磁性納米顆粒:合成、表面修飾及其在藥物傳遞中的應(yīng)用
1. 介紹
磁性納米顆粒(MNP)由于其生物相容性、易于表面修飾和磁性等特性,在生物醫(yī)學(xué)和工業(yè)應(yīng)用中得到了廣泛的關(guān)注。磁性納米顆??梢砸远喾N方式使用,與一般的納米顆粒非常相似。然而,這些粒子的磁性增加了一個新的維度,在外部磁場的作用下,它們可以被操縱。這一特性開辟了新的應(yīng)用,將藥物附著在磁性顆粒上,利用磁場在體內(nèi)靶向。通常,靶向是通過附著一個分子來識別另一個特定于期望目標(biāo)區(qū)域的分子來實現(xiàn)的。這通常需要一種化學(xué)識別機制,但并不像設(shè)計的那樣成功。因此,磁性納米顆??梢蕴峁┮环N將藥物運送到人體所需部位的解決方案。磁性納米顆粒雖然可能含有其他元素,但通常是氧化鐵。最常見的氧化鐵有磁鐵礦(Fe3O4)、磁赤鐵礦(γ-Fe2O3)、赤鐵礦(α-Fe2O3)和地長石。根據(jù)實驗條件的不同,可以形成一種或多種氧化鐵相。仔細(xì)控制實驗條件以確保單相的存在是非常重要的。
在應(yīng)用中經(jīng)常遇到的氧化鐵納米顆粒是超順磁性的。超順磁性是磁性的一種形式,可以用小的鐵磁性或鐵磁性納米顆粒觀察到。在足夠小的納米顆粒中,磁化可以在溫度的影響下隨機翻轉(zhuǎn)納米顆粒的方向。而超順磁性納米粒子的磁化率要比順磁性納米粒子大得多。超順磁性發(fā)生在具有單一磁疇的納米顆粒中,即由單一磁疇組成。在這種情況下,可以認(rèn)為納米粒子的磁化是一個單巨磁矩,即納米粒子原子所攜帶的所有單個磁矩的總和。當(dāng)外部磁場作用于超順磁性納米粒子時,它們傾向于沿著磁場排列,導(dǎo)致凈磁化。然而,在沒有外部磁場的情況下,偶極子是隨機定向的,并且沒有凈磁化。最近研究了由多元醇非水均相溶液合成的Fe3O4納米顆粒磁性能的大小依賴性(Caruntu等人,2007)。在前面提到的氧化鐵中,磁鐵礦和磁鐵礦是超順磁性的,這些研究將主要集中在作為磁性納米顆粒的研究中。
本文綜述了近年來對磁性納米顆粒的毒性研究,以說明磁性納米顆粒的生物相容性。利用MNPs進行靶向藥物的研究是非常有前途的,并給出了一些實例。熱療是一種利用磁場提高溫度并導(dǎo)致細(xì)胞死亡的腫瘤的補充治療方法,可以使用MNPs實現(xiàn),并介紹了該領(lǐng)域的一些最新進展。最后,表格總結(jié)了用于藥物輸送應(yīng)用的不同類型的MNP矩陣。
2. 毒性
磁性納米顆粒在生物醫(yī)學(xué)應(yīng)用中引起興趣的主要原因之一是它們的生物相容性。由于這些顆粒被用作藥物傳遞載體,因此應(yīng)詳細(xì)研究它們的細(xì)胞毒性。在體外和體內(nèi)的一些研究表明,這些顆粒對人體具有低毒性。鐵通常通過轉(zhuǎn)鐵蛋白運輸,它可以結(jié)合細(xì)胞表面定位的轉(zhuǎn)鐵蛋白受體。在細(xì)胞質(zhì)中,大部分的細(xì)胞質(zhì)鐵儲存在一種叫做鐵蛋白的特殊蛋白質(zhì)中。由于鐵的生理相關(guān)性,MNPs最初被認(rèn)為是無細(xì)胞毒性的。MNPs可以被自然分解,從而釋放出三鐵,然后參與正常的鐵代謝。然而,人們已經(jīng)認(rèn)識到,MNPs的小尺寸可能會造成額外的危害,因為這些顆??梢栽诩?xì)胞內(nèi)達到很高的局部濃度,并且通常更難以有效地從體內(nèi)清除(Rivera等人,2010;Chan et al., 2002)。此外,游離鐵與自由基的形成有關(guān),自由基對已經(jīng)被病理過程削弱的神經(jīng)組織尤其有害(Winer et al., 2011)。
值得注意的是,在幾乎所有的研究中,毒性都顯示在一定劑量以上顯著增加。盡管MNPs的高負(fù)荷(100 μg/mL)會引起細(xì)胞毒性,但藥物遞送應(yīng)用所需的濃度通常低于適當(dāng)包被的MNPs的毒性水平(Karlsson等人,2008)。
毒性通常是血清蛋白與MNPs表面結(jié)合的結(jié)果,改變了細(xì)胞所接觸的細(xì)胞培養(yǎng)基的組成(Mahmoudi等,2009)。包被的納米顆粒毒性較低,這不僅是因為包被具有生物相容性,還因為介質(zhì)中蛋白質(zhì)、離子和其他成分的吸附位點較低(Mahmoudi et al., 2010)。
Figure 1. A) Schematic representation of the possible exchange/interaction scenarios at the bionanointerface at the cellular level. (B) Schematic drawing of the structure of protein–nanoparticle in blood plasma confirming the existence of various protein binding (e.g. an outerweakly interacting layer of protein (full red arrows) and a hard slowly exchanging corona of proteins (right) (Mahmoudi et al., 2011)
磁鐵礦(Fe3O4)和磁鐵礦(γ-Fe2O3)由于具有氧化/還原反應(yīng)的能力而表現(xiàn)出不同的細(xì)胞反應(yīng)。事實上,與磁鐵礦相比,在沒有降低細(xì)胞活力的情況下,在A549人肺上皮細(xì)胞系中,磁鐵礦已被證明會導(dǎo)致更高水平的氧化性DNA損傷(使用彗星測定法),因為磁鐵礦具有氧化的潛力(Karlsson等人,2009;Karlsson et al., 2008)。
其中一個最敏感的毒性參數(shù)是納米顆粒的表面涂層。表面覆蓋的程度被認(rèn)為是細(xì)胞攝取的主要參數(shù),因為不完全的表面覆蓋被證明可以促進調(diào)理作用和快速內(nèi)吞作用,而完全包裹的MNPs可以逃脫調(diào)理作用,從而延長血漿半衰期(Jung et al., 1995)。帶負(fù)電荷的未包被MNPs已被證明在一定閾值以上表現(xiàn)出細(xì)胞毒性。在體內(nèi)研究中,未包被的MNPs也具有低溶解度,這導(dǎo)致它們在水介質(zhì)中沉淀,阻礙血管。為了降低MNPs的毒性,使用了不同的涂層。H?feli等人(H?feli等人,2009)用聚氧化物(PEO)三嵌段共聚物(PEO- cooh -PEO)涂覆MNPs,發(fā)現(xiàn)PEO尾部嵌段長度與毒性呈負(fù)相關(guān)。PEO尾巴長度在2 kDa以上,適合在體內(nèi)應(yīng)用。Mahmoudi等人(Mahmoudi等人,2009)表明,未包覆的顆粒比包覆聚乙烯醇(PVA)的磁鐵礦顆粒具有更大的毒性。他們還表明,用表面飽和的未包覆顆粒取代,可以顯著降低未包覆顆粒的毒性。用二巰基琥珀酸(DMSA)包覆磁鐵礦顆粒幾乎可以消除這些顆粒的毒性(Auffan et al., 2006),防止顆粒與人皮膚成纖維細(xì)胞直接接觸。然而,在另一項使用DMSA包被的磁鐵礦顆粒的研究中,開發(fā)了一個可量化的細(xì)胞系統(tǒng)模型,并表明即使是中等水平的MNPs在細(xì)胞間傳遞也可能對細(xì)胞功能產(chǎn)生不利影響(Pisanic et al., 2007)。將磁鐵礦顆粒涂覆聚乙烯亞胺(PEI)-聚乙二醇(PEG),并將其毒性與支化PEI涂層進行比較(Schweiger et al., 2011)。引入聚乙二醇被證明具有屏蔽作用,并導(dǎo)致較低的毒性。Lee等人(Lee等人,2011)使用乙二醇雙層穩(wěn)定的磁鐵礦納米顆粒,并證明這些納米顆粒無毒。磁鐵礦顆粒的聚乙二醇涂層也被證明可以降低毒性(Zhou et al., 2011)。
當(dāng)MNPs被嵌入殼聚糖中以獲得磁性殼聚糖顆粒時,由于MNPs被殼聚糖完全覆蓋,它們表現(xiàn)出相對較低的細(xì)胞毒性(Park等,2005)。
雖然葡聚糖是一種復(fù)雜的支鏈葡萄糖,經(jīng)常用于醫(yī)療應(yīng)用,但葡聚糖包被的磁鐵礦顆粒與未包被的磁鐵礦顆粒造成的細(xì)胞死亡一樣多(Berry et al., 2003)。相反,在一項比較研究中,研究了未包被的磁鐵礦、未包被的磁鐵礦、葡聚糖包被的磁鐵礦和葡聚糖包被的磁鐵礦的細(xì)胞毒性,兩種樣品的細(xì)胞毒性均未低于100 mg/mL,唯一顯示遺傳毒性的樣品是葡聚糖包被的磁鐵礦(Singh et al., 2012)。在更廣泛的研究中,Ding et al. (Ding et al., 2010)發(fā)現(xiàn)右旋糖酐雜交磁鐵礦納米顆粒的細(xì)胞毒性具有細(xì)胞特異性。提示對相關(guān)細(xì)胞的毒性評價應(yīng)予以關(guān)注。
磁性顆粒周圍的一系列次級表面活性劑已經(jīng)在體內(nèi)進行了毒性測試。檸檬酸和褐藻酸表面活性劑的毒性明顯低于淀粉、癸酸和聚乙二醇。這項研究顯示了優(yōu)化表面涂層以最小化毒性的重要性(Kuznetsov et al., 1999)。
在一項體內(nèi)研究中,白蛋白包被的磁鐵礦微球被證明具有良好的耐受性(Kuznetsov等人,1999年)。與單劑量的阿霉素相比,含有阿霉素(一種抗癌藥物)的磁鐵礦白蛋白微球?qū)游锲鞴倩蚣?xì)胞的毒性降低,顯著減少了副作用(Ma等人,2000年)。然而,在另一項研究中,白蛋白衍生的MNPs被發(fā)現(xiàn)會導(dǎo)致膜破壞,可能是由于蛋白質(zhì)與膜脂肪酸和磷脂的相互作用(Berry et al., 2003)。
在細(xì)胞水平上評估了Fe3O4-PLLA-PEG-PLLA(聚l -乳酸)顆粒與未包覆磁鐵礦顆粒相比的低細(xì)胞毒性。它們還在分子水平上產(chǎn)生低基因毒性和免疫毒性。急性毒性試驗顯示其毒性相當(dāng)?shù)?,因此在生物醫(yī)學(xué)應(yīng)用方面具有很大的潛力(Chen等人,2012年)。包裹在MPEG-PLGA (PLGA:聚乳酸-羥基乙酸)膠束中的磁鐵礦沒有細(xì)胞毒性(Ding et al., 2012)。
最近,粒徑而非包覆度被認(rèn)為是影響巨噬細(xì)胞攝取速率的主要因素(Raynal et al., 2004)。在體內(nèi)研究中,使用了50 nm(葡聚糖包覆)和4 μm(聚苯乙烯包覆)的MNPs,并證明其在眼內(nèi)應(yīng)用是安全的(Raju等,2011)??诜?、靜脈注射和腹腔注射約20 nm的MNPs沒有表現(xiàn)出毒性(zeng et al., 2005)。含有1,6己二胺的MNPs經(jīng)大鼠腦內(nèi)或動脈內(nèi)接種后顯示是安全的(Muldoon等,2005)。40 nm的MNPs對mES細(xì)胞無毒(Shundo et al., 2012)。
在磁場作用下,MNPs顯示出更高的毒性,導(dǎo)致細(xì)胞死亡(Simioni et al., 2007;Bae et al., 2011)。這是腫瘤治療的基礎(chǔ),熱療,這將在后面的章節(jié)中詳細(xì)總結(jié)。
盡管MNPs是常規(guī)使用,但長期影響和潛在的神經(jīng)毒性尚未得到廣泛評估(Yildirimer et al., 2011)。
磁性納米顆粒具有較低的細(xì)胞毒性,因此在生物醫(yī)學(xué)領(lǐng)域的應(yīng)用引起了科學(xué)界對將其用作藥物載體的極大興趣。在給藥方面,主要有兩個目標(biāo);首先是將藥物靶向到體內(nèi)所需的區(qū)域,以減少對其他器官的副作用;其次是藥物的控制釋放,以避免經(jīng)典的過量/不足周期。磁性納米顆粒可能為這兩個目標(biāo)提供解決方案。磁性納米顆粒周圍的涂層經(jīng)過優(yōu)化,可以像大多數(shù)納米顆粒一樣以所需的方式攜帶和釋放藥物。然而,這些粒子的獨特性質(zhì)是它們是磁性的,允許使用外部磁場來操縱。這形成了磁性靶向的基礎(chǔ),其中攜帶藥物的磁性顆粒在施加磁場時被定向到特定區(qū)域。
3.磁定位
為了在體外研究磁靶向,建立了一個模擬腫瘤供血分支動脈的實驗裝置,使其參數(shù)接近真實系統(tǒng)。實現(xiàn)了粒子的靶向,并發(fā)現(xiàn)其依賴于分支點的磁性體積力(Gitter et al., 2011)。利用相同的設(shè)置,構(gòu)建了結(jié)合特征點磁體積力、磁體位置和定量數(shù)據(jù)的新型定量定位圖。高達97%的納米顆粒被成功地靶向到所選的分支中(Gitter et al., 2011)。研制了一種磁性靶向給藥系統(tǒng)(MT-DDS)裝置,該裝置通過控制超導(dǎo)磁體產(chǎn)生的磁場強度和/或梯度,實現(xiàn)了藥物在體內(nèi)局部病變部位的導(dǎo)航和積累。將Mn-Zn鐵氧體顆粒注入實驗裝置中,形成y形玻璃管的靜脈模型。這是磁靶向藥物輸送系統(tǒng)的一項基本技術(shù),可在循環(huán)器官系統(tǒng)的血管中提供藥物導(dǎo)航,這顯示了MT-DDS藥物輸送方法的實用性(Mishima et al., 2007)。
為了驗證MNP在血管中的植入,并將注射的MNP靶向到這些特定的部位,我們建立了實驗和計算模型。為了產(chǎn)生強大的局部場梯度,使用改進的軟光刻技術(shù)構(gòu)建了嵌入磁錨的微流體通道來分析捕獲過程。實驗調(diào)查的定性結(jié)果證實了該方法的合法性。研究表明,在血管系統(tǒng)的特定點捕獲和聚集磁性微球是可能的(Forbes et al., 2003)。
理論模擬和實驗證明了利用兩種磁源局部靶向給藥是一種優(yōu)化高濃度磁性載體遞送到人體特定部位的新方法。實驗結(jié)果表明,在與人體冠狀動脈的尺寸和流速相一致的流動條件下,在相當(dāng)高的濃度下捕獲直徑為微米和亞微米的超順磁珠是可能的。同樣的實驗用非磁性網(wǎng)進行,結(jié)果沒有明顯的捕獲,這表明植入物負(fù)責(zé)提供必要的磁場梯度和力來捕獲注射的微珠(Yellen et al., 2005)。
磁性靶向的體內(nèi)研究有幾項。磁性殼聚糖納米顆粒成功靶向腫瘤組織進行光動力治療,導(dǎo)致其在皮膚和肝臟組織中的低積累(Sun et al., 2009)。
磁性碳納米管(MNT)的內(nèi)表面有一層磁鐵礦納米顆粒,其中化療藥物摻入孔中。通過使用外部放置的磁鐵將藥物基質(zhì)引導(dǎo)到局部淋巴結(jié),MNTs可以在引流的靶向淋巴結(jié)中保留數(shù)天并持續(xù)釋放化療藥物(Yang et al., 2008)。
在一項體外研究中,含有抗癌藥物的磁性聚(乙基-2-氰基丙烯酸酯)(PECA)納米顆粒被證明在外磁場下釋放藥物并具有磁性遷移率(Yang et al., 2006)。
與靜脈注射相比,在頸動脈內(nèi)注射聚乙烯亞胺(PEI)修飾的磁性納米顆粒并結(jié)合磁性靶向治療可使顆粒的腫瘤夾閉率增加30倍(Chertok等人,2010)。
采用磁鐵礦-葡聚糖復(fù)合顆粒給藥米托蒽醌。磁性靶向治療后,腫瘤組織中的米托蒽醌濃度始終顯著升高,磁鐵應(yīng)用后血漿鐵濃度下降,表明磁性靶向治療的有效性(Krukemeyer et al., 2012)。
在另一項研究中,米托蒽醌與超順磁性fe3o4納米顆粒結(jié)合,載藥納米顆粒通過靠近腫瘤的股動脈給藥。在應(yīng)用過程中,磁性納米顆粒被聚焦的外部磁場吸引到腫瘤上。高效液相色譜-生物分布實驗的結(jié)果表明,與常用的全身應(yīng)用相比,磁性藥物靶向可以使治療劑在所需的身體隔室(即腫瘤區(qū)域)的富集程度提高50倍(Alexiou等,2011)。
在體外研究了由磁鐵礦羧基改性聚二乙烯基苯和含磁鐵礦組成的磁性納米粒子種子在植入體輔助磁性藥物靶向(IA-MDT)中的應(yīng)用。在70mT的外部磁場下,首先從通過70%多孔聚合物支架的流體中捕獲MNP種子,該支架設(shè)計用于模擬毛細(xì)血管組織。然后將其用于捕獲具有相同磁場的磁性藥物載體顆粒(MDCPs) (Mangual等,2011)。
聚[苯胺-co- n -(1- 1-丁酸)苯胺](SPAnH)用1,3-雙(2-氯乙基)-1-亞硝基脲(BCNU)包覆Fe3O4顆粒。結(jié)合- bccu -3可以在體外和體內(nèi)通過外部施加的磁鐵集中在目標(biāo)部位。當(dāng)應(yīng)用于腦腫瘤時,發(fā)現(xiàn)磁靶向可以增加結(jié)合的bccnu -3的濃度和保留率(Hua et al., 2011)。
磁性靶向增強了膠質(zhì)肉瘤中淀粉包被的超順磁性納米顆粒的積累,并通過磁共振成像進行了量化(Chertok等人,2008)。
聚乙二醇改性交聯(lián)淀粉包覆磁鐵礦顆粒的體內(nèi)磁靶向研究。在9l -膠質(zhì)瘤大鼠模型中證實了靜脈注射PEG-MNPs的選擇性,增強腦腫瘤靶向性。腫瘤靶向結(jié)果是很有希望的,并為進一步開發(fā)載藥PEG-MNPs和同時優(yōu)化所使用的磁靶向策略提供了依據(jù)(Cole et al., 2011)。
制備了由磁性Fe3O4 (SHMNPs)核和水穩(wěn)定自摻雜聚[N-(1-一丁酸))苯胺(SPAnH)殼組成的超高磁化納米載體(SHMNCs),具有高阿霉素(DOX)載藥量(27.1% wt%)。這些納米載體增強了藥物的熱穩(wěn)定性,并最大限度地提高了通過磁靶向治療給藥MGH-U1膀胱癌細(xì)胞的效率,部分原因是避免了p糖蛋白(P-gp)泵提高細(xì)胞內(nèi)DOX濃度的作用(Hua等,2011)。
磁性顆粒也可以瞄準(zhǔn)腫瘤區(qū)域,因此可以對腫瘤進行成像。氧化鐵顆粒常被用作MRI造影劑。事實上,磁鐵礦是FDA批準(zhǔn)的造影劑。由于本研究的重點是對磁性給藥技術(shù)做一個全面的總結(jié),因此本文將不涉及用于MRI的磁性顆粒。
從上述研究中可以看出,磁靶向是一種將藥物靶向到所需區(qū)域(通常是腫瘤)的有效方法。然而,在一些研究中,與磁靶向同時使用的還有靶向配體。在沒有磁性靶向的情況下,靶向是利用藥物載體上的配體特異性結(jié)合靶區(qū)域的受體來實現(xiàn)的。用于此目的的常見配體是葉酸(或葉酸)。葉酸對葉酸受體蛋白有很高的親和力,葉酸受體蛋白通常在許多人類癌癥的表面表達。如果將葉酸標(biāo)記在攜帶藥物的納米顆粒上,葉酸就會與癌細(xì)胞表面的葉酸受體結(jié)合,并通過內(nèi)吞作用攝取綴合物,完成靶向藥物遞送。磁性粒子與靶配體的示意圖如圖2所示。
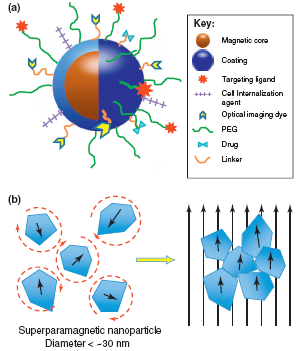
Figure 2. a) Schematic representation of the ‘‘core–shell’’ structure of MNPs and multi-functional surface decoration. MNPs consist of a magnetic iron oxide core coated with a biocompatible material (e.g. polysaccharide, lipid, protein, small silane linkers, etc.). Functional groups on the surface of coatings are often used to link ligands for molecular targeting, cellular internalization, optical imaging, enhanced plasma residence and/or therapy. The variety of moieties that decorate the MNP surface imparts the nanoparticle with its multi-functional, theranostic character. (b) Illustration of superparamagnetic MNP response to applied magnetic fields. MNPs comprise rotating crystals that align with the direction of an applied magnetic field. Crystal reorientation provides the high magnetic susceptibility and saturation magnetization observed for this material. The circular dashed lines around the superparamagnetic nanoparticles on the left illustrate the randomization of their orientation, due to temperature effects, in the absence of a magnetic field. (Cole et al.2011).
以生物相容性Pluronic F127和與葉酸(FA)化學(xué)偶聯(lián)的聚乳酸(F127- pla)共聚物為載體,以超順磁性氧化鐵顆粒為基礎(chǔ)合成磁性納米載體。磁顆粒在外加磁場的作用下被引導(dǎo)到靶部位,從而提高抗腫瘤藥物的治療效果。這些定性結(jié)果都是通過簡單的統(tǒng)計分析進行的,這說明雙重靶向機制可以帶來更好的治療效果(Huang et al., 2012)。
超順磁性氧化鐵納米晶體和DOX與膽固醇(含或不含葉酸)共封裝到PLGA/聚合脂質(zhì)體核殼納米載體中。在體外實驗中,葉酸靶向DOX負(fù)載磁性核殼納米載體對Hela細(xì)胞的靶向效果優(yōu)于非葉酸靶向載體(Wang et al., 2012)。
熱敏磁性脂質(zhì)體具有DPPC:膽固醇:DSPE-PEG2000:DSPE-PEG2000-葉酸(DPPC:雙棕櫚酰磷脂酰膽堿;DSPE: 1,2-二硬脂酰-sn-甘油-3-磷酸乙醇胺),摩爾比為80:20:45:0.5。與Caelyx?(一種市售的多柔比星脂質(zhì)體制劑)、非磁性葉酸靶向脂質(zhì)體(FolDox)和表達葉酸受體的腫瘤細(xì)胞系(KB和HeLa細(xì)胞)中的游離DOX相比,該載體通過永久磁場物理靶向腫瘤細(xì)胞時,DOX的細(xì)胞攝取顯著增加(Pradhan等,2010)。
制備了具有介孔二氧化硅核殼結(jié)構(gòu)的磁性納米顆粒,并成功地以熒光聚合物鏈作為標(biāo)記段,葉酸作為癌癥靶向段,裝載藥物進行定向釋放。藥物載體被證明能夠鉆入細(xì)胞膜并獲得抗癌藥物持續(xù)釋放到細(xì)胞質(zhì)中。體外細(xì)胞對藥物的攝取表明,載藥納米復(fù)合材料可以有效靶向腫瘤細(xì)胞(Chen et al., 2010)。
合成了具有熒光SiO2外殼的Fe3O4納米顆粒,并與葉酸共軛的超支化聚甘油(聚丙烯酰胺接枝Fe3O4@SiO2納米顆粒)接枝。體外研究表明,與巨噬細(xì)胞和成纖維細(xì)胞相比,人卵巢癌細(xì)胞(SKOV-3)對葉酸結(jié)合納米顆粒的攝取明顯優(yōu)先(Wang等,2011)。
磁鐵礦納米顆粒通過吸附微粒表面的聚合層(羧甲基殼聚糖)進行修飾,并與熒光染料、靶向配體和藥物分子結(jié)合,以改善靶向特異性診斷和可能的治療應(yīng)用。將丙烯酸、葉酸、顆粒(Fe3O4-CMC-AA-FA)和DOX加載到MNPs的外殼中,并在不同ph下進行釋放研究。與NIH3T3細(xì)胞相比,F(xiàn)e3O4-CMC-AA-FA-DOX NPs對HeLa細(xì)胞的生長有明顯的抑制作用,且呈劑量依賴性。該研究表明,F(xiàn)e3O4-CMC-AA-FA能夠提供單一納米級結(jié)構(gòu),具有腫瘤細(xì)胞靶向、成像和藥物遞送功能。這是首次描述基于殼聚糖的MNPs系統(tǒng)具有上述所有功能(Sahu et al., 2012)。
葉酸以外的其他配體也被用于納米顆粒的活性靶向。DOX在5-羧基熒光素(FAM)標(biāo)記的AGKGTPSLETTP肽(A54)偶聯(lián)淀粉包被氧化鐵納米顆粒上證明了DOX負(fù)載的A54- ions (SION:超順磁性氧化鐵)對BEL-7402細(xì)胞的特異性。顯微鏡圖像證明,在體內(nèi)外磁場作用下,負(fù)載dox的A54-SIONs成功靶向裸鼠腫瘤組織(Yang et al., 2009)。
配體修飾CPT-SAIO@SiO2納米載體用于遞送抗癌劑(包封喜樹堿(CPT))。研究發(fā)現(xiàn),修飾后的納米載體對CPT具有相當(dāng)高的載藥效率,并且通過網(wǎng)格蛋白介導(dǎo)的內(nèi)吞作用被過表達EGFR的癌細(xì)胞攝取。通過外部磁刺激釋放CPT分子在細(xì)胞內(nèi)被證明在技術(shù)上是成功的,并且確保了比使用游離藥物獲得更高的治療效果(Tung et al., 2011)。
西妥昔單抗-抗表皮生長因子受體(EGFR)單克隆抗體與聚乙二醇-聚(ε-己內(nèi)酯)(PEG-PCL)連接的免疫膠束。這些膠束負(fù)載DOX和Fe3O4超順磁性氧化鐵。結(jié)果表明,免疫膠束比其非靶向?qū)?yīng)物更有效地抑制細(xì)胞增殖。西妥昔單抗免疫膠束更有效地與過度表達表皮生長因子受體的癌細(xì)胞結(jié)合,導(dǎo)致更多的超順磁性氧化鐵和DOX被轉(zhuǎn)運到這些細(xì)胞中(Liao et al., 2011)。
通過ph敏感鍵將抗癌藥物偶聯(lián)到聚乙二醇化的SPIO (SPIO:超順磁性氧化鐵)納米載體上。腫瘤靶向配體,環(huán)(arg - gly - asp - d - ph - cys) (c(RGDfC))肽,PET 64Cu螯合劑,大環(huán)1,4,7-三氮雜環(huán)壬烷- n, N0, n00 -三乙酸(NOTA),被偶聯(lián)到PEG臂的遠端。體外實驗表明,與不含crgd的SPIO納米載體相比,結(jié)合crgd的SPIO納米載體具有更高的細(xì)胞攝取水平。這些納米載體在聯(lián)合靶向抗癌藥物遞送和PET/MRI腫瘤雙模成像方面表現(xiàn)出了很好的特性(Yang等,2011)。
聚脂質(zhì)體(PEG/RGD-MPLs);由兩親性聚合物十八烷基季銨化改性聚γ-谷氨酸(OQPGA)、聚乙二醇化OQPGA、RGD肽接枝OQPGA和磁性納米顆粒組成。它提供了一種對具有超順磁特性的外部永磁體產(chǎn)生響應(yīng)的可能性,當(dāng)用于體內(nèi)磁性組織靶向時。細(xì)胞攝取結(jié)果表明,PEG/RGDMPLs(含RGD和磁性顆粒)在MCF-7細(xì)胞中比非RGD和非磁性載體表現(xiàn)出更多的藥物細(xì)胞攝取(Su et al., 2012)。
這些研究表明,磁靶向是一種有效的將藥物靶向腫瘤區(qū)域的方法。結(jié)合使用合適配體的主動靶向,配體修飾的載藥磁性納米顆粒在外部磁場的作用下提供了有效的藥物靶向系統(tǒng)。一旦將磁性顆粒靶向到所需區(qū)域,最常用的腫瘤治療方法之一就是熱療。當(dāng)磁性納米顆粒在腫瘤附近并受到交變磁場作用時,會使腫瘤散發(fā)熱量并升高腫瘤溫度,導(dǎo)致腫瘤細(xì)胞死亡。
4. 高熱治療
溫度在40°C到45°C之間通常被稱為熱療。溫度高達42℃可使癌細(xì)胞更容易受到輻射的影響,并導(dǎo)致一定程度的細(xì)胞凋亡,而溫度在45℃以下被稱為熱消融,并導(dǎo)致細(xì)胞直接殺傷(壞死)(Elsherbini等人,2011)。在磁性納米粒子熱療治療癌癥的臨床應(yīng)用中,在保護正常組織的同時確保對腫瘤的最大損傷是非常重要的(saloum et al., 2009)。磁性納米粒子熱療在癌癥治療中具有很大的潛力,但由于預(yù)期的加熱分布難以控制,導(dǎo)致腫瘤組織溫度升高不均勻和不充分,嚴(yán)重限制了其應(yīng)用。組織中顆粒的運輸包括載體溶液的細(xì)胞外運輸、載體溶液中顆粒的運輸以及顆粒與細(xì)胞表面的相互作用等過程。納米顆粒在腫瘤中的細(xì)胞外轉(zhuǎn)運尚不清楚(saloum et al., 2008)。
熱療幾乎總是與其他形式的癌癥治療一起使用,如放射治療和化療。熱療可能會使一些癌細(xì)胞對輻射更敏感,或傷害輻射無法傷害的其他癌細(xì)胞。當(dāng)熱療和放射治療同時進行時,它們通常在一小時內(nèi)進行。熱療還可以增強某些抗癌藥物的作用(Van der Zee, 2002;Wust et al., 2002)。
許多臨床試驗研究了熱療與放射治療和/或化療的結(jié)合。這些研究集中在多種癌癥的治療上,包括肉瘤、黑色素瘤、頭頸癌、腦癌、肺癌、食道癌、乳腺癌、膀胱癌、直腸癌、肝癌、闌尾癌、子宮頸癌和腹膜內(nèi)膜癌(間皮瘤)(Falk等人,2001;Feldman et al., 2003;Chang et al., 2001)。這些研究中的許多,但不是全部,都表明當(dāng)熱療與其他治療相結(jié)合時,腫瘤大小顯著減小。然而,并非所有這些研究都表明,接受聯(lián)合治療的患者生存率提高(Van der Zee, 2002;Wust et al., 2002)。磁性納米材料在熱療基礎(chǔ)和聯(lián)合治療中的獨特優(yōu)勢如圖3所示。
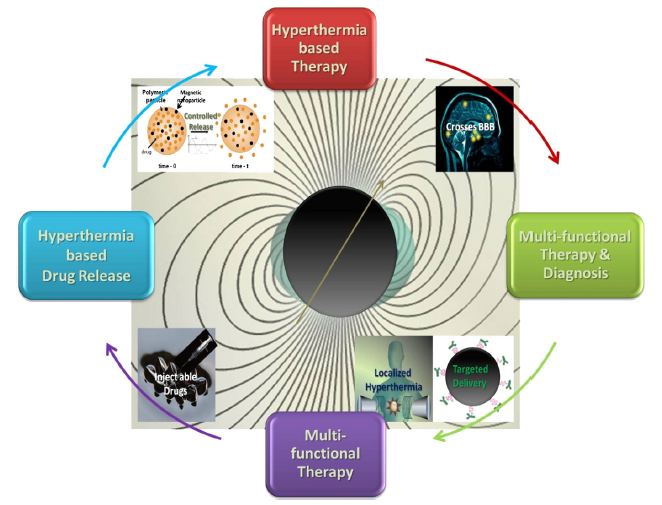
Figure 3. A schematic representation of some of the unique advantages of magnetic nanomaterials for hyperthermia-based therapy and controlled drug delivery (Kumar & Mohammad, 2011).
用于加熱的交變磁場中的磁損失是由于粒子系統(tǒng)中磁化反轉(zhuǎn)的不同過程引起的:(1)磁滯,(2)nsamel或Brown弛豫,(3)粘性懸浮液中的摩擦損失(Hergt et al., 2006)。
超順磁性納米粒子的磁化能在熱能的作用下自發(fā)改變?nèi)∠?。磁化強度在兩個平衡位置之間振蕩。兩個方向變化之間的典型時間由n 弛豫時間給出,其中τ0是一個嘗試時間,其值約為10?9-10?10秒。在沒有磁場的情況下,溶液中的磁性納米顆粒會隨機運動,這種運動被稱為布朗運動。當(dāng)磁場作用于流體中的磁性納米顆粒時,由于磁場與磁化相互作用產(chǎn)生的扭矩,磁性納米顆粒旋轉(zhuǎn)并逐漸與磁場對齊。磁性納米粒子與一個小的外部磁場對齊所花費的時間由布朗弛豫時間給出,其中η為溶劑粘度。磁場旋轉(zhuǎn)和磁化旋轉(zhuǎn)之間的延遲導(dǎo)致磁滯。磁滯回線的面積作為熱能在環(huán)境中耗散,用于磁熱療。
當(dāng)交變磁場(AMF)作用于磁性材料時,由于磁滯,一種稱為比吸收率(SAR)的能量被耗散,并以納米粒子的W/g表示。
給定材料的SAR由SAR = Af給出,其中a為磁滯回線面積,f為磁場交變頻率。A用J/g表示,也稱為材料的“比損耗”,因此在一些研究中也可以將SAR稱為比損耗功率(specific Loss Power, SLP)。
幾個研究小組對同一材料估計的SAR值可能會有所不同,因為它取決于幾個參數(shù),如載體流體的物理和化學(xué)性質(zhì)、涂層材料、施加磁場的頻率和振幅、Fe3O4納米顆粒的大小和形狀(Elsherbini等,2011)。
通過優(yōu)化一種算法,可以反向確定多個納米顆粒注入引起的最佳加熱模式,從而開發(fā)出以A為單位的優(yōu)化SAR分布(saloum et al., 2009)。對于熱療應(yīng)用,需要高SAR值。實現(xiàn)這一目標(biāo)的一種方法是增加磁場強度,但平均磁場強度應(yīng)保持在30 mT以下,以避免形成渦流,從而誘發(fā)毒性(alphandsamry et al., 2012)。
SPA作為粒徑函數(shù)的研究表明,構(gòu)成加熱劑的納米顆粒的平均粒徑和粒徑分布是設(shè)計高效加熱納米顆粒的核心參數(shù)(Goya et al., 2008)。
不幸的是,很難對顆粒的組成和大小進行直接比較。在一項研究中,制備了多疇鐵氧體顆粒,并將其與有右旋糖酐包覆和未包覆的小磁鐵礦顆粒的SAR數(shù)據(jù)進行了比較。大鐵氧體顆粒(200-400 nm)的每質(zhì)量功率吸收比相同成分的小顆粒要低得多,盡管兩種顆粒尺寸分布都相對較寬(Jordan等,1993)。然而,大尺寸的顆粒無法到達細(xì)胞內(nèi)部(Martín-Saavedra et al., 2010)。
通過對Pluronic F127包覆的Fe3O4單分散顆粒進行量熱測量,結(jié)果表明,在給定頻率下,超順磁顆粒的升溫速率取決于顆粒大小,這與早期的理論預(yù)測一致。結(jié)果還表明,正如預(yù)測的那樣,SLP隨著樣本多分散性而擴大(Gonzales-Weimuller et al., 2009)。
同樣,單疇尺寸范圍(20-70 nm)的平均粒徑與小尺寸分布寬度相結(jié)合可以增強SLP (Hergt et al., 2007)。
先前的研究表明,在靶向磁熱療治療中,組織鐵濃度與加熱速率之間存在線性關(guān)系(Pardoe et al., 2003)。動脈栓塞熱療(AEH)的一個關(guān)鍵組成部分是鐵磁顆粒在正常肝實質(zhì)(NHP)以及腫瘤組織中的濃度和分布。如果NHP中的顆粒分布是不均勻的,有高濃度的區(qū)域,那么在AEH期間可能會導(dǎo)致不需要的壞死區(qū)域(Moroz等人,2002)。
在另一項研究中,幾種具有代表性的磁性氧化鐵納米顆粒用于不同的制備方法(濕化學(xué)沉淀法、研磨法、細(xì)菌合成法、磁性粒度分餾法)進行比較研究(Hergt et al., 2006)。市面上可買到的非常小的超順磁顆粒被認(rèn)為不是有效加熱腫瘤的最佳材料。相反,超順磁性磁鐵礦納米顆粒被證明適合于用射頻誘導(dǎo)埃利希腫瘤熱療(Elsherbini et al., 2011)。
在超順磁狀態(tài)下,單個粒子的磁矩與MNPs的SPA值之間沒有相關(guān)性。盡管SPA機制與初始疇壁之間的關(guān)系仍有待確定,但最佳粒徑建議接近Fe3O4相從單疇到多疇轉(zhuǎn)變的臨界尺寸(Goya et al., 2008)。
當(dāng)使用磁性納米顆粒作為磁顆粒熱療的熱源時,了解顆粒是否在組織液中自由移動或固定在腫瘤組織上是特別有趣的。固定狀態(tài)決定了所給粒子的弛豫行為,從而決定了它們的比加熱功率(Dutz et al., 2011)。如果粒子不能旋轉(zhuǎn),由布朗弛豫引起的溫度升高可以忽略不計。有研究表明,羧甲基右旋糖酐包被的磁性顆粒注射入腫瘤后,對腫瘤組織的固定作用相當(dāng)強(Dutz et al., 2011)。
研究了nsamel弛豫對無法移動或旋轉(zhuǎn)的磁性納米粒子的影響,并比較了線性和圓極化場中的損耗(De chaltel et al., 2009)。在低于拉莫爾頻率的頻率下,線性極化是更好的熱電源,在高頻率下(超過拉莫爾頻率),圓極化是更好的。如果各向同性樣品中的nsamel松弛是主要機制,則無需考慮在復(fù)雜幾何結(jié)構(gòu)中產(chǎn)生圓極化場的技術(shù)復(fù)雜性。
為了使組織中顆粒濃度達到所需溫度,MNP的比熱功率(SHP)應(yīng)盡可能高。發(fā)現(xiàn)超順磁粒子尺寸的比熱功率與外加交變磁場的頻率和振幅的關(guān)系符合弛豫理論的預(yù)測。對于較小的平均尺寸(約6納米),加熱能力小得可以忽略不計,而較大的顆粒提供適合熱療的加熱(Gl?ckl等人,2006年)。
文獻中通常報道的SLP數(shù)據(jù)顯示,當(dāng)場幅為10 kA m-1,頻率約為400 kHz時,散射的數(shù)量級為10 - 100w g-1 (Hergt et al., 2006)。
總而言之:
為了在小腫瘤(目前直徑小于10mm)中達到有效的治療溫度,MNP的SLP必須大大增加。
MPH的主要實際問題是腫瘤的MNP供應(yīng)不足。對于IT注射,組織中MNP分布的不均勻性可能導(dǎo)致局部溫度差異,從而無法區(qū)分熱療和熱消融。由于腫瘤部分溫度增強不足,存在存活腫瘤細(xì)胞增殖的風(fēng)險。
對于MNP的全身供應(yīng)(例如抗體靶向),MNP的靶富集必須大大增強以達到治療溫度。特別是,小靶點(低于目前診斷限度的轉(zhuǎn)移)的治療似乎是一個值得懷疑的希望(Hergt等人,2007)。
對熱療有用的比損失功率受到交變電場振幅和頻率的嚴(yán)重限制。在400 kHz和10 kA m?1條件下,對于平均粒徑約為18 nm的粒子,如果粒徑分布足夠窄,其SLP值可達數(shù)百W g?1。對于磁鐵礦晶體平均直徑約為35 nm的細(xì)菌磁小體,發(fā)現(xiàn)了接近1 kW g?1的非常大的SLP值(Hergt et al., 2006)。
生物醫(yī)學(xué)中常用的氨基硅烷修飾的MNPs,細(xì)菌磁小體(BM)在AMF下表現(xiàn)出更好的加熱效果。雖然這兩種顆粒都被發(fā)現(xiàn)通過使用相同濃度的MNPs和磁小體熱療來增強細(xì)胞活力的降低,但腦轉(zhuǎn)移瘤需要更低強度的電流才能在腫瘤細(xì)胞中產(chǎn)生類似的抑制作用(Liu et al., 2012)。
當(dāng)磁小體鏈(由蛋白質(zhì)組成的細(xì)絲相互結(jié)合)在癌細(xì)胞存在的情況下孵育,并暴露在頻率為198 kHz、平均磁場強度為20或30 mT的交變磁場中時,它們能有效抑制癌細(xì)胞的增殖。這種行為可以解釋為高細(xì)胞內(nèi)化,溶液中良好的穩(wěn)定性和磁小體鏈的均勻分布,從而實現(xiàn)高效加熱(alphandsamry et al., 2012)。
當(dāng)磁小體鏈被加熱時,將磁小體結(jié)合在一起的細(xì)絲發(fā)生變性,得到個體磁小體,這些磁小體在交變磁場作用下容易聚集,在溶液中不穩(wěn)定,不能有效抑制癌細(xì)胞增殖(alphand 等人,2012)。
聚乙二醇甲基丙烯酸甲醚和二甲基丙烯酸甲酯與氧化鐵作為植入式生物材料。結(jié)果表明,可以通過改變AMF強度來控制水凝膠的溫度,使凝膠達到高溫(42-45℃)或熱燒蝕(60-63℃)溫度。水凝膠納米復(fù)合材料達到的最終溫度可以根據(jù)這些溫度范圍中的任何一個進行定制。水凝膠在AMF中加熱,加熱響應(yīng)取決于凝膠中的氧化鐵負(fù)載和磁場強度(Meenach et al., 2010)。
采用脂質(zhì)薄膜法制備了含有磁流體和光敏劑基配合物(CB:ZnPc-ML)的陽離子磁性脂質(zhì)體。這一結(jié)果表明,光和交流磁場一起應(yīng)用比單獨應(yīng)用兩種處理更有效(Bolfarini et al., 2012)。
用載藥PCPG磁脂質(zhì)體評價磁熱療聯(lián)合化療的效果。熱敏藥物釋放在磁場的影響下發(fā)生,這種聯(lián)合治療被證明比單獨治療更有效(Kulshrestha et al., 2012)。
結(jié)果表明,即使吸收量相同,鐵磁MNPs獲得的溫度也高于超順磁MNPs獲得的溫度。這是由于磁滯損耗和磁松弛之間的熱效率差異。熱的產(chǎn)生主要是由于磁滯損耗而不是磁弛豫。與細(xì)胞外產(chǎn)生的熱量相比,納米顆粒進入細(xì)胞并吸附在細(xì)胞膜上產(chǎn)生的熱量對細(xì)胞的破壞至關(guān)重要(Baba et al., 2012)。
根據(jù)一些人的說法,眾所周知的氧化鐵流體變得不受歡迎,因為它們的鐵原子與血紅蛋白的鐵原子很難區(qū)分。一個建議的解決方案是使用混合鐵氧體(MFe2O4,其中M?Co, Mn, Ni, Zn)來具有一系列的磁性能。這些鐵氧體因其節(jié)省時間、固有毒性低、易于合成、物理和化學(xué)穩(wěn)定性以及合適的磁性而受到特別關(guān)注(Sharifi et al., 2012)。
Giri等人研究了100納米以下的檸檬酸鹽包覆鐵氧體顆粒。發(fā)現(xiàn)涂層材料的飽和磁化強度降低,因為磁化強度與相同磁性材料的重量成正比。發(fā)現(xiàn)矯頑力足以使熱療中的遲滯損失加熱。磁滯數(shù)據(jù)表明,這些樣品(涂覆)表現(xiàn)出足夠的磁滯損失,以獲得破壞腫瘤細(xì)胞所需的溫度。
鐵素體顆粒在殼聚糖基質(zhì)中以不同的比例制備(Park等人,2005)。隨著殼聚糖比例的增加,達到熱療所需時間縮短,飽和磁化強度降低。優(yōu)化鐵素體-殼聚糖的比例在熱療方面具有廣闊的應(yīng)用前景。
6-12納米的鈷鈦鐵氧體納米顆粒被證明適用于熱療應(yīng)用(Ichiyanagia等人,2012)。Zn-Gd鐵氧體顆粒是合適的,但如果你用聚乙二醇(PEG)蓋住它們,它們就沒有用了(Yao等人,2009)。與聚(甲基丙烯酸酯)和聚(2-羥乙基甲基丙烯酸酯)共聚的7.5 nm鈷鐵氧體顆粒被證明適用于熱療(Hayashi等人,2012)。CoFe2O4鐵氧體顆粒(鐵磁性)被證明適用于熱療(Skumiel, 2006)。
研究各種材料的納米顆粒產(chǎn)生的加熱,鋇-鐵氧體和鈷-鐵氧體不能產(chǎn)生足夠的MFH加熱,鐵-鈷產(chǎn)生的加熱速度太快而不安全,而fcc鐵-鉑、磁鐵礦和磁鐵礦都能夠產(chǎn)生穩(wěn)定的可控加熱。鐵鈷MNPs誘導(dǎo)的溫度變化太大,而鋇鐵氧體和鈷鐵氧體MNPs不能提供足夠的熱量來治療腫瘤。模擬表明,磁鐵礦、fcc鐵鉑和磁鐵礦MNPs非常適合MFH,可以將腫瘤加熱到41°C以上,同時保持周圍健康組織溫度低于該值(Kappiyoor et al., 2010)。
熱可逆水凝膠(波洛沙姆,殼聚糖),可容納20% w/v的磁性微粒,被證明是不夠的。然而,海藻酸鹽水凝膠含有10% w/v的磁性微粒,外部凝膠化導(dǎo)致植入物定位到腫瘤周圍,而內(nèi)部凝膠化在原位失敗。有機凝膠配方由溶解在單一有機溶劑中的沉淀聚合物組成,顯示出不同的微觀結(jié)構(gòu)。在DMSO (DMSO:二甲基亞砜)中含有40% w/v磁性微粒的8%聚(乙烯-乙烯醇)形成了腫瘤鑄造和熱傳遞方面最合適的植入物(Le Renard等人,2010)。
細(xì)胞培養(yǎng)實驗表明,通過調(diào)整磁性微球MMS的數(shù)量和AMF的暴露時間,可以將磁鐵礦納米顆粒嵌入介孔二氧化硅基質(zhì)中,實現(xiàn)輕度到非常高強度的熱處理(Martín-Saavedra et al., 2010)。對含鐵的10-40納米多壁碳納米管的加熱效果進行了研究,并證明是合適的(Krupskaya et al., 2009)。
幾種磁流體被證明適合熱療應(yīng)用。在一項比較研究中,對16種商業(yè)磁流體進行了調(diào)查,并區(qū)分了最合適的磁流體(Kallumadil et al., 2009)。20-30μm的磁鐵礦微膠囊嵌入瓊脂體中,在交變磁場下產(chǎn)生熱量(Miyazaki et al., 2012)。氣凝膠基質(zhì)中10納米的磁鐵礦納米顆粒是潛在的熱療劑,其中氣凝膠基質(zhì)可用于聯(lián)合治療的藥物裝載(Lee et al., 2012)。
從上述研究中可以看出,雖然對于理想的熱療磁性材料并沒有一個明確的定義,但根據(jù)具體情況,可以采用幾種材料。藥物傳遞與熱療聯(lián)合治療在該領(lǐng)域具有廣闊的應(yīng)用前景。
磁性藥物載體
由于磁性顆粒具有低毒性和靶向性,因此它們是藥物遞送的潛在候選者,通常將磁性顆粒涂覆以穩(wěn)定其抗沉淀,確保其低細(xì)胞毒性并在基質(zhì)中攜帶藥物。
在體內(nèi)應(yīng)用中,SPION顆粒應(yīng)被包裹以防止藥物分子偶聯(lián)并限制與非靶向細(xì)胞側(cè)的相互作用,防止顆粒團聚并增強藥物裝載和釋放。SPION涂層的不同方法導(dǎo)致不同的聚合物組裝如圖4所示。在多糖包衣和共聚物包衣中,所得到的顆粒呈均勻的包芯狀。在另一種涂覆方法中,聚合物分子固定在磁性顆粒表面,形成刷狀結(jié)構(gòu)。脂質(zhì)體和膠束形成的分子形成核殼結(jié)構(gòu),核中有磁性粒子。這些結(jié)構(gòu)可用于保留疏水區(qū)域的藥物包封。(Veiseh et al., 2010)。
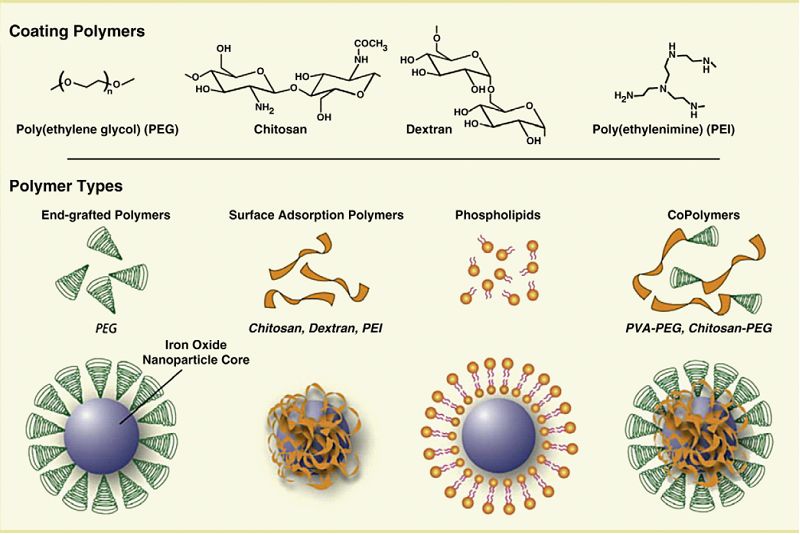
不同的顆粒被設(shè)計為藥物傳遞載體,表1給出了這些顆粒的總結(jié)。
表1 磁性納米材料在藥物傳遞中的應(yīng)用綜述
Type of magnetic nanoparticle | Particle size | Coating agent | Drug | Design matrix | Drug release mechanism | Ref |
Fe3O4 |
Core diameter of 10 - 15 nm Final diameter of 160 nm |
chitosan/PAA multilayer | cefradine |
Layer-by-Layer (LBL) The drug molecules were entrapped inside the hollow spheres through diffusion process |
pH responsive | Zhang et al., 2006 |
Fe3O4 | Final diameter of "/>1 μm | sodium carboxy methyl cellulose and chitosan | ------ | self-assembly shell composed of layers of carboxy methyl cellulose and chitosan around the magnetic core | ------------ | Cui et al., 2011 |
Fe3O4 |
Core diameter of 8 nm Final diameter of of 107 nm |
chitosan | cefradine |
cross-linking the particles with glutaraldehyde and the drug is embedded in the polymer matrix |
pH responsive | Li et al., 2007 |
Fe3O4 |
Core diameter of 5 nm Final diameter of 1–1.5cm |
Alginate / chitosan | insulin | insulin encapsulation in alginate/chitosan beads. The beads containing insulin were prepared in triplicate by extrusion method. | Magnetic field | Finotelli et al., 2010 |
Fe3O4 | Final diameter of 200 nm | multiwalled carbon nanotubes (MWNTs) | doxorubicin | The MWNT-hybrid nanocomposites provided an efficient way for the extraction and enrichment of doxorubicin via π–π stacking of DOX molecules onto the polyaromatic surface of MWNTs. | pH responsive | Shen et al., 2011 |
Fe3O4 | Core diameter of 3 nm | CNTs | --------- | Magnetic nanoparticles adsorb on the CNT ends | ------ | Panczyk et al., 2010 |
Fe3O4 |
Core diameter of 5–10 nm. CNTs average diameter of about 30–50 nm and average length of about 100–500 nm |
CdTe QDs and CNTs | ------- |
CNT-SPIO-CdTe nanohybrids via LBL assembly |
---------- | Chen et al., 2010 |
γ-Fe2O3 | Core diameter of 10 nm | CNT | Diaminophenothiazine (methylene blue) | monodisperse, inherently open-ended, multi-wall CNTs loaded with magnetic iron-based nanoparticles that are encapsulated within the tube graphitic walls | Vermisoglou et al., 2011 | |
Fe3O4 |
Core diameter of 8–12 nm mACs had a mean diameter of about 30 nm MWNTs=40-60 nm |
(mMWNTs) and magnetic-activated carbon particles (mACs) |
gemcitabine (GEM) | Fe3O4 nanoparticles are on the outer surface of the PAA functionalized MWNTs and the drug is adsorbed on the surface . | Yang et al., 2011 | |
CoFe2O4 nanoparticles |
Core diameter of 6 nm MWCNTs with an outer diameter of 10–30 nm and an average length of 0.5–2 μm |
MWCNT/cobalt ferrite (CoFe2O4) magnetic hybrids | doxorubicin |
cobalt ferrite is on the outer surface of the MWCNT |
pH responsive | Wu et al., 2011 |
γ-Fe203 |
Core diameter of 5 nm Final diameter of 100 nm |
DNA | fluorescein | Single-stranded DNA was immobilized onto the silica network, and the magnetic particles are loaded onto the network. The complementary DNA sequence was then attached to magnetic nanoparticles. | Temperature responsive | Ruiz-Hernandez et al., 2011 |
Fe3O4 |
Core diameter of of 8 nm Final diameter of 150 nm |
PEG-functionalized porous silica shell | doxorubi-cin | DOZ conjugated magnetite particles are coated with silica to obtain core/shell nanoparticles and the whole composite is coated with PEG |
the breaking of the bonding of the drug to the carrier or the swelling and degradation of the polymer. |
Chen et al., 2010 |
α-Fe2O3 |
Core diameter of 13 nm micron-sized mesoporous molecular sieves (with 2.9-nm pores) MCM-41 and MCM-48 powders gave mean pore sizes of 3.7 and 3.5 nm, a size between 1 and 4 μm. and hollow silica microcapsules (pores of 2.7, average diameter being around 3 μm. and 15 nm. 250-nm wall thickness |
hollow silica microcapsules | --------- | Magnetic particles are encapsulated inside the hollow silica microcapsules | ------------ | Arruebo et al., 2006 |
Fe3O4 |
Core diameter of 10 nm Final diameter of 100 nm with 20 nm silica shell |
SiO2@ Fe3O4 core–shell NPs |
Silica-magnetite nanocomposites are emulsified and self-assembly of magnetic-mesoporous heteronanorods at the interface of water-in-oil droplets takes place. |
Zhang et al., 2011 | ||
Fe3O4 |
Particles between 150nm and 4.5 μm |
silica,arabic acid and cross-linked polysaccharide |
antibody | particles with starch derivative or polymeric arabic acid as matrix material functionalized with an antibody | --- | Sieben et al., 2001 |
Fe3O4 |
Final diameter if 202 nm |
β-cyclodextrin and pluronic polymer (F-127) | curcumin |
multi-layer polymer coating around the magnetic particle and the drug is encapsulated via diffusion into polymer matrix |
The initial burst of release was due to immediate dissociation of surface bound curcumin molecules that exist on the CD or F127 polymer matrix. The remaining sustained drug release was due to the slow release of the drug entrapped inside CD and/or F127 polymer layers. |
Yallapu et al., 2011 |
Fe3O4 | Core diameter of 14.8 nm |
2-hydroxypropyl-cyclodextrin (HCD) onto the gum arabic modified magnetic nanoparticles (GAMNP) |
ketoprofen | polymers grafted onto magnetic particles(Multilayer polymer matrix) |
drug molecules are rapidly released from HCDGAMNP, whereas some remains associated to degredation of HCD-GAMNP |
Banerjee & Chen, 2009 |
Fe3O4 |
Final diameter of 13 nm. |
(3-aminopropyl) triethoxysilane coated (APTES-MNPs) with b-cyclodextrin (β-CD). | ------- |
layer-by-layer |
--------- | Cao et al., 2009 |
Fe3O4 | Core diameter of 9.2 nm | Oleic acid, sodium dodecyl benzene sulfonate SDBS, bovine serum albumin (BSA) | --- | Oleic acid capped magnetic nanoparticles are embedded in the SDBS micelle and BSA adsorbs onto the micellar entity. | ---- | Yang et al., 2009 |
Fe3O4 | Final diameter of 300 nm |
poly (N-isopropylacrylamide) PNIPAAm and poly(D,L-lactide-co-glycolide) PLGA |
Bovine serum albumin (BSA) and curcumin |
(MLNPs) with a magnetic core and two shells made up of temperature-sensitive polymers (PNIPAAm) were encapsulated with PLGA. BSA was first loaded into PNIPAAm magnetic nanoparticles. Second, curcumin was loaded to PLGA to form the multilayer nanoparticles | Temperature responsive | Koppolu et al., 2010 |
Fe3O4 | Final diameter of 150 nm | dextran | fluorescein (Fluo) or TEXAS RED? (Texas) fluorescent dye |
By oxidizing Ferumoxides (FE) (suspension consisting of dextran- coated SPION) hydroxyl groups on the dextran coating are oxidized to aldehyde groups. Lysine fixable fluorescein (Fluo) or TEXAS RED? (Texas) fluorescent dye (supplied as lysine fixable dextran conjugates) was reacted with aldehyde FE and the fluorescent dye is conjugated to FE SPION (FL FE). |
---- | Lee et al., 2008 |
Fe3O4 |
Core diameter of 5 5 nm Final diameter of 4 μm, |
Fe3O4/ PAH |
fluorescein isothiocyanate (FITC)-Dextran |
layer-by-layer (LbL) assembly FITC-dextran nanoparticle is coated with PSS polyelectrolyte which contains the magnetic particles forming a magnetic shell around the particle. |
Magnetic field | Hu et al., 2008 |
Fe3O4 | Core diameter of 12 nm | coated with starch, dextran, PEG or MPEG | ---- | Polymeric networks cover a large number of continuous magnetic monodomains. | ---- | Huong et al., 2009 |
magnetic fluids Carboxyde-xtran coated DDM128 P6 (dextran–magnetite) Aminosilane coated (aminosilane–magnetite) MFL AS |
DDM128 P6: core diameter of 3 nm MFL AS: core diameter of 15 nm. |
dextran- or aminosilane-coated | ---- | ----- | Jordan et al., 2006 | |
Fe3O4 | Core diameter of 7 nm | PVA and starch | PVA coated particles as large clusters where starch coated ones are be densely dispersed in the polymeric matrix | Voit et al., 2001 | ||
Fe3O4 | Final diameter of 110±22 nm | starch | ------ |
Core-shell particles |
----- | Chertok et al., 2008 |
Fe3O4 |
coated with starch (G100) particles final diameter of 110 (±22) nm gumarabic polysaccha-ride Matrix (Gara) particles final diameter of 189nm Final diameter 225 nm after PEI addition |
Polyethyleneimine (PEI) | -------- | Surface modification of carboxyl-bearing Gara nano particles with PEI | -------- | Chertok et al., 2010 |
Fe3O4 |
Final diameter of (140-190 nm) |
Aminated, cross-linked starch and aminosilane coated Fe3O4 modified with PEG | To ensure that cross-linked starch particles was functionally similar to aminosilane coated particles, starch particles were covalently strengthened and aminated with concentrated ammonia to form aminated-precursor (DN). PEG is then linked to aminated precursors, DN and aminosilane particles with N-Hydroxysuccinimide (NHS) chemistry. | Cole et al., 2011 | ||
Fe3O4 | Core diameter of 4-10 nm |
PVA and PVA with partially exchanged carboxyl groups. |
---- | ----- | Lee et al., 1996 | |
Fe3O4 | Core diameter of 10 nm | PVA matrix | --- | the films of 200 mm depth and different concentrations of iron oxide particles in the PVA matrix. | ---- | Novakova et al., 2003 |
Fe3O4 |
Core diameter of 5–10 nm Final diameter of 108-155 nm |
PVA | ---- | core-shell, all iron-oxide particles surrounded by a layer of PVA polymer. | ---- | Qui & Winnik, 2000 |
γ-Fe203 |
Core diameter of 14, 19 and 43 nm Final particles are of diameter 43 nm |
PNIPAM | doxorubicin | MNP cluster is coated with PNIPAM and the nanoparticl is dehydrated. Core shell morphology is achieved with dispersion free-radical polymerization | Thermoresponsive | Purushotham et al., 2009 |
Fe3O4 | core diameter of 13 nm | PNIPAM | doxorubicin | Core shell morphology by dispersion polymerization where drug loaded PNIPAM shell contains magnetite clusters. | Thermoresponsive | Purushotham et al., 2010 |
Fe3O4 |
Core diameter of 11.21 nm Final particles are of diameter less than 250 μm |
PMMA |
fluorescein isothiocyanate (FITC) |
Thermoresponsive | Urbina et al., 2008 | |
γ-Fe203 |
Core diameter of 20 nm Final particles are of diameter 400 nm |
carbon | doxorubicin | Drug is released form the surface of on-coated or partially coated magnetic particles |
released from the surface of our particles at a slow rate via desorption |
Ibarra et al., 2007 |
Fe3O4 | Final particles are of diameter ~10–20 nm |
poly[aniline-co-sodium N-(1-onebutyric acid)] aniline (SPAnNa) |
1,3-bis(2-chloroethyl)-1- nitrosourea |
Microcapsule nanoparticles are encapsulated during the aggregation, forming the Fe3O4/SPAnH nanoparticles | Ultrasound and externally applied magnetic field. | Chen et al., 2010 |
Fe3O4 |
Core diameter of 8 nm Final particles of diameter 5.2 μm |
PEs: poly(styrene sulfonate) (PSS, Mw~70000) and poly(allylamine hydrochloride) (PAH, Mw~50000). |
Melamine formaldehyde microparticle is coated with polyelectrolytes (PE) in a layer-by-layer (LbL) assembly by solvent controlled precipitation of PE. The core is then dissolved and nanoparticles are infiltrated into the capsule core. | Gaponik et al., 2004 | ||
Fe3O4 | Final particle diameter of 300–1300 nm |
polystyrene |
Similar technique to abovementioned method. | Madani et al., 2011 | ||
Fe3O4 |
Core diameter of 13 nm Final particle diameter of 3 μm |
poly(sodium 4-styrenesulfonate) (PSS) and poly(allylamine hydrochloride) (PAH) |
Dye |
Similar technique to abovementioned method. |
Magnetic heating | Katagiri et al., 2010 |
Fe3O4 |
Core diameter of 20 nm Final particle diameter of 2.82 μm |
(PDDA/PSS)2/PDDA |
Dye | Similar technique to abovementioned method. | Magnetic heating | Katagiri et al., 2011 |
Fe3O4 and γ-Fe203 |
Fe3O4 and γ-Fe203 core diameters of 9.5 and 4.3 nm, respectively |
Ca alginate beads | ----- | The nanoparticles were entrapped in Ca alginate beads, “egg-box like” structure of Ca alginate | ------- | Finotelli et al., 2005 |
Fe3O4 | Particle diameter of 58 nm | NP aggragates in humic acid (HA) | --- | HA adsorbs onto magnetite particles | ------ | Hu et al., 2010 |
Fe3O4 | Final particle diameter of 7.5 nm | amino silane( 3-aminopropyl triethoxysilane) | --- |
nearly monolayer coating of amino silane on the magnetite particle surface |
--- | Ma et al., 2003 |
Fe3O4 |
Core particle diameter of 10–15 nm Final particle diameter of 400±80 nm |
poly-L-lysine hydrochloride (PLL), poly-L-glutamic acid (PGA) | DNA |
layer-by-layer (LbL) assembly on polycarbonate templates with subsequent removal of these templates. In the inner surface of polycarbonate templates, first poly-L-lysine hydrochloride (PLL) and poly-L-glutamic acid (PGA) are absorbed linking by electrostatic interactions as a polyelectrolyte layer. Then, multi polyelectrolyte layers are assembled on polycarbonate membrane and Fe3O4 nanoparticles are linked to PLL layer as Fe3O4/PLL bilayers. |
----------- | He et al., 2008 |
γ-Fe203 |
Core diameters of 12 nm. Final particle diameter of 35 nm (PEI) and 46 nm (PEI plus PEO-PGA) |
Poly(ethylene imine) and Poly(ethylene oxide)-block-poly(glutamic acid) |
--- | MNPs stabilized with polymers in two layer-by-layer deposition steps. | ---- | Thunemann et al., 2006 |
Fe3O4 | Core diameter of 12 nm |
aminosilane coating |
--- | ---- | --- | Maier-Hauff et al., 2011 |
Fe3O4 and γ-Fe203 |
Core diameters of 10 nm Final particle diameter of 96 ±15 nm |
poly(ethylene glycol) (PEG) | doxorubicin | one-pot synthesis of colloids of SPION-DOX-PEG particles, PEG shell reduces the access of cellular enzymes to the drug-particle linkage and thus limits and/or delays the anticancer effect. |
specific release mechanism for drug delivery is enzymatic cleavage, however the PEG shell seems to reduce the access of cellular enzymes to the drug-particle linkage and thus limits and/or delays the anticancer effect. |
Shkilnyy et al., 2010 |
本文綜述了磁性納米顆粒在藥物傳遞中的應(yīng)用。磁性納米顆粒由于其生物相容性、低毒性和在磁場作用下可操縱的能力而引起了人們的極大興趣。這些特殊的性質(zhì)允許它們被用作藥物載體,通過直接將藥物附著在顆粒上,或者通常通過使用天然或合成聚合物來幫助攜帶藥物并將磁性顆粒嵌入聚合物基質(zhì)中。已經(jīng)探索了幾種類型的藥物和涂層作為藥物載體,表1總結(jié)了非常有限的選擇。這些顆粒表面修飾的便利性為靶向部分附著在顆粒表面提供了機會,從而促進了靶向。磁性納米顆粒的靶向主要是在施加外部磁場的情況下進行的,該磁場作為一種外力將顆粒定位在體內(nèi)所需的區(qū)域。一旦磁性粒子靠近腫瘤,對它們施加交變磁場,就會導(dǎo)致介質(zhì)溫度上升到42攝氏度,這是熱療所需的溫度,熱療是與化療和放療一起進行的補充治療。我們相信,在不久的將來,這些迷人的粒子將在現(xiàn)有的基礎(chǔ)上獲得更大的成功,并找到進一步的潛在應(yīng)用。
參考文獻
1. AlexiouC.TietzeR.SchreiberE.JurgonsR.RichterH.TrahmsL.RahnH.OdenbachS.LyerS.2011Cancer therapy with drug loaded magnetic nanoparticles-magnetic drug targeting, Journal of Magnetism and Magnetic Materials, 32314041407
2. AlphandéryE.GuyotF.ChebbiI.2012Preparation of chains of magnetosomes, isolated from Magnetospirillum magneticum strain AMB-1 magnetotactic bacteria, yielding efficient treatment of tumors using magnetic hyperthermia, International Journal of Pharmaceutics, 434444452
3. ArrueboM.GalanM.NavascuesN.TellezC.MarquinaC.IbarraM. R.SantamariaJ.2006Development of Magnetic Nanostructured Silica-Based Materials as Potential Vectors for Drug-Delivery Applications, Chemistry of Materials, 1819111919
4. AuffanM.DecomeL.Ros,eJ.OrsiereT.De MeoM.BrioisV.2006In vitro interactions between DMSA-coated maghemite nanoparticles and human fibroblasts: a physicochemical and cyto-genotoxical study, Environmental Science & Technology, 4043674373
5. BabaD.SeikoY.NakanishiT.ZhangH.ArakakiA.MatsunagaT.OsakaT.2012Effect of magnetite nanoparticles on living rate of MCF-7 human breast cancer cells, Colloids Surf B Biointerfaces, 95254257
6. BaeJ.E.HuhM.I.RyuB.K.DoJ.Y.JinS.U.MoonM.J.JungJ.C.ChangY.KimE.ChiS.G.LeeG.H.ChaeK.S.2011The effect of static magnetic fields on the aggregation and cytotoxicity of magnetic nanoparticles, Biomaterials, 3294019414
7. BanerjeeS. S.ChenD.H.2009Cyclodextrin-conjugated nanocarrier for magnetically guided delivery of hydrophobic drugs, Journal of Nanoparticle Research, 1120712078
8. BerryC. C.WellsS.CharlesS.CurtisA. S.2003Dextran and albumin derivatised iron oxide nanoparticles: in?uence on ?broblasts in vitro, Biomaterials, 24455457
9. BolfariniG. C.Siqueira-MouraM. P.DemetsG. J. F.MoraisP. C.TedescoA. C.2012In vitro evaluation of combined hyperthermia and photodynamic effects using magnetoliposomes loaded with cucurbit[7]uril zinc phthalocyanine complex on melanoma, Journal of Photochemistry and Photobiology B: Biology, DOI:10.1016/j.jphotobiol.2012.05.009,impress.
10. CaoH.HeJ.DengL.GaoX.2009Fabrication of cyclodextrin-functionalized superparamagnetic Fe3O4/amino-silane core-shell nanoparticles via layer-by-layer method, Applied Surface Science, 25579747980
11. CaruntuD.CaruntuG.O’ConnorC. J.2007Magnetic properties of variable-sized Fe3O4 nanoparticles synthesized from non-aqueous homogeneous solutions of polyols, Journal of Physics D: Applied Physics, 4058015809
12. ChanW. C. W.MaxwellD. J.GaoX. H.BaileyR. E.HanM. Y.NieS. M.2002Luminescent QDs for multiplexed biological detection and imaging, Current Opinion in Biotechnology, 134046
13. ChangE.AlexanderH. R.LibuttiS. K.HurstR.ZhaiS.FiggW. D.BartlettD. L.2001Laparoscopic continuous hyperthermic peritoneal perfusion, Journal of the American College of Surgeons, 193225229
14. ChenA.Z.LinX.F.WangS.B.LiL.LiuY.G.YeL.WangG.Y.2012Biological evaluation of Fe3O4-poly(l-lactide)-poly(ethylene glycol)-poly(l-lactide) magnetic microspheres prepared in supercritical CO2, Toxicology Letters, 2127582
15. ChenB.ZhangH.ZhaiC.DuN.SunC.XueJ.YangD.HuangH.ZhangB.XiecQ.WuY.2010Carbon nanotube-based magnetic-fluorescent nanohybrids as highly efficient contrast agents for multimodal cellular imaging, Journal of Materials Chemistry, 2098959902
16. ChenD.JiangM.LiN.GuH.XuQ.GeJ.XiaX.LuJ.2010Modification of magnetic silica/iron oxide nanocomposites with fluorescent polymethacrylic acid for cancer targeting and drug delivery, Journal of Materials Chemistry, 2064226429
17. ChenF.H.ZhangL.M.ChenQ.T.ZhangY.ZhangZ.J.2010Synthesis of a novel magnetic drug delivery system composed of doxorubicin-conjugated Fe3O4 nanoparticle cores and a PEG-functionalized porous silica Shell, Chemical Communications, 4686338635
18. ChenP.Y.LiuH.L.HuaM.Y.YangH.W.HuangC.Y.ChuP.C.LyuL.A.Tseng-CI.FengL.Y.TsaiH.C.ChenS.M.LuY.J.WangJ.J.YenT.C.MaY.H.WuT.ChenJ.P.ChuangJ.I.ShinJ.W.HsuehC.WeiK.C.2010Novel magnetic/ultrasound focusing system enhances nanoparticle drug delivery for glioma treatment, Neuro-Oncology, 1210501060
19. ChertokB.DavidA. E.YangV. C.2010Polyethyleneimine-modified iron oxide nanoparticles for brain tumor drug delivery using magnetic targeting and intra-carotid administration, Biomaterials, 3163176324
20. ChertokB.MoffatB. A.DavidA. E.YuF.BergemannC.RossB. D.YangV. C.2008Iron Oxide Nanoparticles as a Drug Delivery Vehicle for MRI Monitored Magnetic Targeting of Brain Tumors, Biomaterials, 29487496
21. ColeA. J.DavidA. E.WangJ.GalbánC. J.HillH. L.YangV. C.2011Polyethylene glycol modified, cross-linked starch-coated iron oxide nanoparticles for enhanced magnetic tumor targeting, Biomaterials, 3221832193
22. ColeA. J.DavidA. E.WangJ.GalbánC. J.YangV. C.2011Magnetic brain tumor targeting and biodistribution of long-circulating PEG-modified, cross-linked starch-coated iron oxide nanoparticles, Biomaterials, 3262916301
23. ColeA. J.YangV. C.DavidA. E.2011Cancer theranostics: the rise of targeted magnetic nanoparticles, Trends in Biotechnology, 29323332
24. CuiM.WangF.J.ShaoZ.Q.LuF.S.WangW.J.2011Influence of DS of CMC on morphology and performance of magnetic microcapsules, Cellulose, 1812651271
25. De ChatelP. F.NándoriI.HaklJ.MészárosS.VadK.2009Magnetic particle hyperthermia: Néel relaxation in magnetic nanoparticles under circularly polarized field, Journal of Physics: Condensed Matter, 21124202124210
26. DingJ.TaoK.LiJ.SongS.SunK.2010Cell-specific cytotoxicity of dextran-stabilized magnetite nanoparticles, Colloids and Surfaces B: Biointerfaces, 79184190
27. DingG.GuoY.LvY.LiuX.XuL.ZhangX.2012A double-targeted magnetic nanocarrier with potential application in hydrophobic drug delivery, Colloids and Surfaces B: Biointerfaces, 916876
28. DutzS.KetteringM.HilgerI.MüllerR.ZeisbergerM.2011Magnetic multicore nanoparticles for hyperthermia--influence of particle immobilization in tumor tissue on magnetic properties, Nanotechnology, 22265102265109
29. ElsherbiniA. A.SaberM.AggagM.El -ShahawyA.ShokierH. A.2011Magnetic nanoparticle-induced hyperthermia treatment under magnetic resonance imaging, Magnetic Resonance Imaging, 29272280
30. FalkM. H.IsselsR. D.2001Hyperthermia in oncology, International Journal of Hyperthermia, 17118
31. FeldmanA. L.LibuttiS. K.PingpankJ. F.BartlettD. L.BeresnevT. H.MavroukakisS. M.SteinbergS. M.LiewehrD. J.KleinerD. E.AlexanderH. R.2003Analysis of Factors Associated with Outcome in Patients with Malignant Peritoneal Mesothelioma Undergoing Surgical Debulking and Intraperitoneal Chemotherapy, Journal of Clinical Oncology, 2145604567
32. FinotelliP. V.DaSilva. D.Sola-PennaM.RossiA. M.FarinaM.AndradeL. R.TakeuchiA. Y.Rocha-LeaoM. H.2010Microcapsules of alginate/chitosan containing magnetic nanoparticles for controlled release of insulin, Colloids and Surfaces B: Biointerfaces, 81206211
33. FinotelliP. V.SampaioD. A.MoralesM. A.RossiA. M.Rocha-Le?oM. H.2005Ca Alginate as Scaffold for Iron Oxide Nanoparticles Synthesis, 2nd Mercosur Congress on Chemical Engineering, 4th Mercosur Congress on Process Systems Engineering, Rio de Janeiro- Brazil.
34. ForbesZ. G.YellenB. B.BarbeeK. A.FriedmanG.2003An Approach to Targeted Drug Delivery Based on Uniform Magnetic Fields, IEEE Transactions on Magnetics, 3933723377
35. GaponikN.RadtchenkoI. L.SukhorukovG. B.RogachA. L.2004Luminescent Polymer Microcapsules Addressable by a Magnetic Field, Langmuir, 2014491452
36. GitterK.OdenbachS.2011Experimental investigations on a branched tube model in magnetic drug targeting, Journal of Magnetism and Magnetic Materials, 32314131416
37. GitterK.OdenbachS.2011Quantitative targeting maps based on experimental investigations for a branched tube model in magnetic drug targeting, Journal of Magnetism and Magnetic Materials, 32330383042
38. Gl?cklG.HergtR.ZeisbergerM.DutzS.NagelS.WeitschiesW.2006The effect of field parameters, nanoparticle properties and immobilization on the specific heating power in magnetic particle hyperthermia, Journal of Physics: Condensed Matter, 18S2935S2949
39. Gonzales-WeimullerM.ZeisbergerM.KrishnanK. M.2009Size-dependant heating rates of ironoxide nanoparticles for magnetic fluid hyperthermia, Journal of Magnetism and Magnetic Materials, 32119471950
40. GoyaG. F.LimaE.Jr ArelaroA. D.TorresT.RechenbergH. R.RossiL.MarquinaC.IbarraM. R.2008Magnetic Hyperthermia with Fe3O4 Nanoparticles: The Influence of Particle Size on Energy Absorption, IEEE Transactions on Magnetics, 4444444447
41. H?feliU. A.Rif?eJ. S.Carmichael-BaranauskasA.Harris-ShekhawatL.MarkF.DaileyJ. P.BardensteinD.2009Cell Uptake and in vitro Toxicity of Magnetic Nanoparticles Suitable for Drug Delivery, Molecular Pharmaceutics, 614171428
42. HayashiK.MaedaK.MoriyaM.SakamotoW.YogoT.2012In situ synthesis of cobalt ferrite nanoparticle / polymer hybrid from a mixed Fe-Co methacrylate for magnetic hyperthermia, Journal of Magnetism and Magnetic Materials, 32431583164
43. HeQ.TianY.CuiY.M?hwaldH.LiJ.2008Layer-by-layer assembly of magnetic polypeptide nanotubes as a DNA carrier, Journal of Materials Chemistry, 18748754
44. HergtR.DutzS.2007Magnetic particle hyperthermia-biophysical limitations of a visionary tumor therapy, Journal of Magnetism and Magnetic Materials, 311187192
45. HergtR.DutzS.MüllerR.ZeisbergerM.2006Magnetic particle hyperthermia: nanoparticle magnetism and materials development for cancer therapy, Journal of Physics: Condensed Matter, 18S2919S2934
46. HuJ.D.ZeviY.KouX.M.XiaoJ.WangX.J.JinY.2010Effect of dissolved organic matter on the stability of magnetite nanoparticles under different pH and ionic strength conditions, Science of the Total Environment, 40834773489
47. HuS.H.TsaiC.H.LiaoC.F.LiuD.M.ChenS.Y.2008Controlled Rupture of Magnetic Polyelectrolyte Microcapsules for Drug Delivery, Langmuir, 241181111818
48. HuaM.Y.LiuH.L.YangH.W.ChenP.Y.TsaiR.Y.HuangC.Y.TsengI.C.LyuL.A.MaC.C.TangH.J.YenT.C.WeiK.C.2011The effectiveness of a magnetic nanoparticle-based delivery system for BCNU in the treatment of gliomas, Biomaterials, 32516527
49. HuaM. Y.YangH. W.LiuH. L.TsaiR. Y.PangS. T.ChuangK. L.ChangY. S.HwangT. L.ChangY. H.ChuangH. C.ChuangC. K.2011Superhigh-magnetization nanocarrier as a doxorubicin delivery platform for magnetic targeting therapy, Biomaterials, 3289999010
50. HuangC.TangZ.ZhouY.ZhouX.JinY.LiD.YangY.ZhouS.2012Magnetic micelles as a potential platform for dual targeted drug delivery in cancer therapy, International Journal of Pharmaceutics, 429113122
51. HuongN. T.GiangL. T. K.BinhN. T.MinhL. Q.2009Surface modification of iron oxide nanoparticles and their conjunction with water soluble polymers for biomedical application, Journal of Physics: Conference Series, 187012046012051
52. IbarraM. R.Fernandez-PachecoR.ValdiviaJ. G.MarquinaC.GutierrezM.2007Magnetic Nanoparticle Complexes for Drug Delivery, and Implanted Magnets for Targeting, American Institute of Physics, 89899105
53. IchiyanagiaY.ShigeokaD.HirokiT.MashinoT.KimuraS.TomitakaA.UedaK.TakemuraY.2012Study on increase in temperature of Co-Ti ferrite nanoparticles for magnetic hyperthermia treatment, Thermochimica Acta, 532123126
54. JordanA.ScholzR.Maier-HauffK.van LandeghemF. K. H.WaldoefnerN.TeichgraeberU.PinkernelleJ.BruhnH.NeumannF.ThiesenB.vonDeimling. A.FelixR.2006The effect of thermotherapy using magnetic nanoparticles on rat malignant glioma, Journal of Neuro-Oncology, 78714
55. JordanA.WustP.F?hlingH.JohnW.HinzA.FelixR.1993Inductive heating of ferrimagnetic particles and magnetic fluids: physical evaluation of their potential for hyperthermia, International Journal of Hyperthermia, 95168
56. JungC. W.JacobsP.1995Physical and chemical properties of superparamagnetic iron oxide MR contrast agents: ferumoxides, ferumoxtran, ferumoxsil, Magnetic Resonance Imaging, 13661674
57. KallumadilM.TadaM.NakagawaT.AbeM.SouthernP.PankhurstQ. A.2009Suitability of commercial colloids for magnetic hyperthermia, Journal of Magnetism and Magnetic Materials, 32115091513
58. KappiyoorR.LiangruksaM.GangulyR.PuriI. K.2010The effects of magnetic nanoparticle properties on magnetic fluid Hyperthermia, Journal of Applied Physics, 108094702094708
59. KarlssonH. L.CronholmP.GustafssonJ.MollerL.2008Copper oxide nanoparticles are highly toxic: a comparison between metal oxide nanoparticles and carbon nanotubes, Chemical Research in Toxicology, 2117261732
60. KarlssonH. L.GustafssonJ.CronholmP.MollerL.2009Size dependent toxicity of metal oxide particles--a comparison between nano- and micrometer size, Toxicology Letters, 188112118
61. KatagiriK.ImaiY.KoumotoK.2011Variable on-demand release function of magnetoresponsive hybrid capsules, Journal of Colloid and Interface Science, 361109114
62. KatagiriK.NakamuraM.KoumotoK.2010Magnetoresponsive Smart Capsules Formed with Polyelectrolytes, Lipid Bilayers and Magnetic Nanoparticles, American Chemical Society, 2768773
63. KoppoluB.RahimiM.NattamaS.WadajkarA.NguyenK. T.2010Development of multiple-layer polymeric particles for targeted and controlled drug delivery, Nanomedicine: Nanotechnology, Biology, and Medicine, 6355361
64. KrukemeyerM. G.KrennV.JakobsM.WagnerW.2012Mitoxantrone-iron oxide biodistribution in blood, tumor, spleen and liver-magnetic nanoparticles in cancer treatment, Journal of Surgical Research, 1753543
65. KrupskayaY.MahnC.ParameswaranA.TaylorA.KramerK.HampelS.LeonhardtA.RitschelM.BüchnerB.KlingelerR.2009Magnetic study of iron-containing carbonnanotubes: Feasibility for Magnetic hyperthermia, Journal of Magnetism and Magnetic Materials, 32140674071
66. KulshresthaP.GogoaM.BahadurD.BanerjeeR.2012In vitro application of paclitaxel loaded magnetoliposomes for combined chemotherapy and hyperthermia, Colloids and Surfaces B: Biointerfaces, 9617
67. KumarC. S. S. R.MohammadF.2011Magnetic nanomaterials for hyperthermia-based therapy and controlled drug delivery, Advanced Drug Delivery Reviews, 63789808
68. KuznetsovO. A.BrusentsovN. A.KuznetsovA. A.YurchenkoN. Y.OsipovN. E.BayburtskiyF. S.1999Correlation of the coagulation rates and toxicity of biocompatible ferromagnetic microparticles, Journal of Magnetism and Magnetic Materials, 1948389
69. Le RenardP.E.JordanO.FaesA.Petri-FinkA.HofmannH.RüfenachtD.BosmanF.BucheggerF.DoelkerE.2010The in vivo performance of magnetic particle-loaded injectable, in situ gelling, carriers for the delivery of local hyperthermia, Biomaterials, 31691705
70. LeeE.H.KimC.Y.ChoaY.H.2012Magnetite nanoparticles dispersed within nanoporous aerogels for hyperthermia Application, Current Applied Physics, 2012, doi:10.1016/j.cap.2012.02.017,impress.
71. LeeJ.IsobeT.SennaM.1996Magnetic properties of ultrafine magnetite particles and their slurries prepared via in-situ precipitation, Colloids and Surfaces A: Physicochemical and Engineering Aspects, 109121127
72. LeeJ.H.SchneiderB.JordanK.E.LiuW.FrankJ. A.2008Synthesis of complexable fluorescent superparamagnetic iron oxide nanoparticles (FL SPIONs) and its cell labeling for clinical application, Advanced Material, 2025122516
73. LeeK.J.AnJ.H.ShinJ.S.KimD.H.YooH.S.ChoC.K.2011Biostability of γ-Fe2O3 nanoparticles Evaluated using an in vitro cytotoxicity assays on various tumor cell lines, Current Applied Physics, 11467471
74. LiL.ChenD.ZhangY.DengZ.RenX.MengX.TangF.RenJ.ZhangL.2007Magnetic and fluorescent multifunctional chitosan nanoparticles as a smart drug delivery system, Nanotechnology, 18405102405108
75. LiaoC.SunQ.LiangB.ShenJ.ShuaiX.2011Targeting EGFR-overexpressing tumor cells using cetuximab-immunomicelles loaded with doxorubicin and superparamagnetic iron oxide, European Journal of Radiology, 80699705
76. LiuR.-t.LiuJ.TongJ.-q.TangT.KongW.C.WangX.-w.LiY.TangJ.-t.2012Heating effect and biocompatibility of bacterial magnetosomes as potential materials used in magnetic fluid hyperthermia, Progress in NaturalScience: Materials International, 223139
77. MaJ.ChenD.TianY.TaoK.2000Toxicity of Magnetic Albumin Microspheres Bearing Adriamycin, Journal of Tongji Medical University, 202261262
78. MaM.ZhangY.YuW.ShenH.-y.ZhangH.-q.GuN.2003Preparation and characterization of magnetite nanoparticles coated by amino silane, Colloids and Surfaces A: Physicochemical and Engineering Aspects, 212219226
79. MadaniM.Sharifi-SanjaniN.Faridi-MajidiR.2011Magnetic polystyrene nanocapsules with core-shell morphology obtained by emulsifier-free miniemulsion polymerization, Polymer Science, Ser. A, 53143148
80. MahmoudiM.SantS.WangB.LaurentS.SenT.2011Superparamagnetic iron oxide nanoparticles (SPIONs): Development, surface modification and applications in chemotherapy, Advanced Drug Delivery Reviews, 632446
81. MahmoudiM.SimchiA.ImaniM.MilaniA. S.StroeveP.2009An in vitro study of bare and poly(ethylene glycol)-co-fumarate coated superparamagnetic iron oxide nanoparticles: a new toxicity identification procedure, Nanotechnology, 20225104
82. MahmoudiM.SimchiA.ImaniM.ShokrgozarM. A.MilaniA. S.H?feliU. O.StroeveP.2010A new approach for the in vitro identification of the cytotoxicity of superparamagnetic iron oxide nanoparticles, Colloids and Surfaces B: Biointerfaces, 75300309
83. MahmoudiM.SimchiA.MilaniA. S.StroeveP.2009Cell toxicity of superparamagnetic iron oxide nanoparticles, Journal of Colloid and Interface Science, 336510518
84. Maier-HauffK.UlrichF.NestlerD.NiehoffH.WustP.ThiesenB.OrawaH.BudachV.JordanA.2011Efficacy and safety of intratumoral thermotherapy using magnetic iron-oxide nanoparticles combined with external beam radiotherapy on patients with recurrent glioblastoma multiforme, Journal of Neuro-Oncology, 103317324
85. MangualJ. O.AvilesM. O.EbnerA. D.RitterJ. A.2011In vitro study of magnetic nanoparticles as the implant for implant assisted magnetic drug targeting, Journal of Magnetism and Magnetic Materials, 32319031908
86. Martín-SaavedraF. M.Ruíz-HernándezE.BoréA.ArcosD.Vallet-RegíM.VilaboaN.2010Magnetic mesoporous silica spheres for hyperthermia therapy, Acta Biomaterialia, 645224531
87. MeenachS. A.HiltJ. Z.AndersonK. W.2010Poly(ethylene glycol)-based magnetic hydrogel nanocomposites for hyperthermia cancer therapy, Acta Biomaterialia, 610391046
88. MishimaF.TakedaS.IzumiY.NishijimaS.2007Development of Magnetic Field Control for Magnetically Targeted Drug Delivery System Using a Superconducting Magnet, IEEE Transactions on Applied Superconductivity, 1723032306
89. MiyazakiT.MiyaokaA.IshidaE.LiZ.KawashitaM.HiraokaM.2012Preparation of ferromagnetic microcapsules for hyperthermia using water/oil emulsion as a reaction field, Materials Science and Engineering C, 32692696
90. MorozP.PardoeH.JonesS. K.StPierre. T. G.SongS.GrayB. N.2002Arterial embolization hyperthermia: hepatic iron particle distribution and its potential determination by magnetic resonance imaging, Physics in Medicine and Biology, 4715911602
91. MuldoonL. L.SandorM.PinkstonK. E.NeuweltE. A.2005Imaging, distribution, and toxicity of superparamagnetic iron oxide magnetic resonance nanoparticles in the rat brain and intracerebral tumor, Neurosurgery, 57785796
92. NovakovaA. A.LanchinskayaV.YuVolkovA. V.GendlerT. S.KiselevaT.YuMoskvinaM. A.ZezinS. B.2003Magnetic properties of polymer nanocomposites containing iron oxide nanoparticles, Journal of Magnetism and Magnetic Materials, 258354357
93. PanczykT.WarzochaT. P.CampP. J.2010A magnetically controlled molecular nanocontainer as a drug delivery system: The effects of carbon nanotube and magnetic nanoparticle parameters from monte carlo simulations, The Journal of Physical Chemistry C, 1142129921308
94. PardoeH.ClarkP. R.StPierre. T. G.MorozP.JonesS. K.2003A magnetic resonance imaging based method for measurement of tissue iron concentration in liver arterially embolized with ferromagnetic particles designed for magnetic hyperthermia treatment of tumors, Magnetic Resonance Imaging, 21483488
95. ParkJ.H.ImK.H.LeeS.H.KimD.H.LeeD.Y.LeeY.K.KimK.M.KimK.N.2005Preparation and characterization of magnetic chitosan particles for hyperthermia application, Journal of Magnetism and Magnetic Materials, 293328333
96. PisanicT. R.2ndBlackwellJ. D.ShubayevV. I.FinonesR. R.JinS.2007Nanotoxicity of iron oxide nanoparticle internalization in growing neurons, Biomaterials, 2825722581
97. PradhanP.GiriJ.RiekenF.KochC.MykhaylykO.D?blingerM.BanerjeeR.BahadurD.PlankC.2010Targeted temperature sensitive magnetic liposomes for thermo-chemotherapy, Journal of Controlled Release, 142108121
98. PurushothamS.ChangP. E. J.RumpelH.KeeI. H. C.NgR. T. H.ChowP. K. H.Tan. C. K.RamanujanR. V.2009Thermoresponsive core-shell magnetic nanoparticles for combined modalities of cancer Therapy, Nanotechnology, 20305101305112
99. PurushothamS.RamanujanR. V.2010Thermoresponsive magnetic composite nanomaterials for multimodal cancer therapy, Acta Biomaterialia, 6502510
100. QuiX.-p.WinnikF.2000Preparation and characterization of PVA coated magnetic nanoparticles Chinese journal of polymer science, 18535539
101. RajuH. B.HuY.VedulaA.DubovyS. R.GoldbergJ. L.2011Evaluation of magnetic micro- and nanoparticle toxicity to ocular tissues, PLoS One, 6e17452e17463
102. RaynalI.PrigentP.PeyramaureS.NajidA.RebuzziC.CorotC.2004Macrophage endocytosis of superparamagnetic iron oxide nanoparticles: mechanisms and comparison of ferumoxides and ferumoxtran-10, Investigative Radiology, 395663
103. RiveraG. P.HuhnD.del MercatoL. L.SasseD.ParakW. J.2010Nanopharmacy: Inorganic nanoscale devices as vectors and active compounds, Pharmacological Research, 62115125
104. Ruiz-HernandezE.BaezaA.Vallet-RegiM.2011Smart Drug Delivery through DNA/Magnetic Nanoparticle Gates, American Chemical Society, 512591266
105. SaavedraF. M.Ruíz-HernándezE.BoréA.ArcosD.Vallet-RegíM.VilaboaN.2010Magnetic mesoporous silica spheres for hyperthermia therapy, Acta Biomaterialia, 645224531
106. SahuS. K.MaitiS.PramanikA.GhoshS. K.PramanikP.2012Controlling the thickness of polymeric shell on magnetic nanoparticles loaded with doxorubicin for targeted delivery and MRI contrast agent, Carbohydrate Polymers, 8725932604
107. SalloumM.MaR.ZhuL.2008An in-vivo experimental study of temperature elevations in animal tissue during magnetic nanoparticle hyperthermia, International Journal of Hyperthermia, 24589601
108. SalloumM.MaR.ZhuL.2009Enhancement in treatment planning for magnetic nanoparticle hyperthermia: optimization of the heat absorption pattern, International Journal of Hyperthermia, 25309321
109. chweigerC.PietzonkaC.HeverhagenJ.KisselT.2011Novel magnetic iron oxide nanoparticles coated with poly(ethylene imine)-g-poly(ethylene glycol) for potential biomedical application: Synthesis, stability, cytotoxicity and MR imaging, International Journal of Pharmaceutics 408130137
110. SharifiI.ShokrollahiH.AmiriS.2012Ferrite-based magnetic nanofluids used in hyperthermia applications, Journal of Magnetism and Magnetic Materials, 324903915
111. ShenS.RenJ.ChenJ.LuX.DengC.JiangX.2011Development of magnetic multiwalled carbon nanotubes combined with near-infrared radiation-assisted desorption for the determination of tissue distribution of doxorubicin liposome injects in rats, Journal of Chromatography A, 121846194626
112. ShkilnyyA.MunnierE.HerveK.SouceM.BenoitR.Cohen-JonathanS.LimeletteP.SaboungiM.L.DuboisP.ChourpaI.2010Synthesis and Evaluation of Novel Biocompatible Super-paramagnetic Iron Oxide Nanoparticles as Magnetic Anticancer Drug Carrier and Fluorescence Active Label, Journal of Physical Chemistry C, 11458505858
113. ShundoC.ZhangH.NakanishiT.OsakaT.2012Cytotoxicity evaluation of magnetite (Fe3O4) nanoparticles in mouse embryonic stem cells, Colloids and Surfaces B: Biointerfaces, 97221225
114. SiebenS.BergemannC.LuKbbe. A.BrockmannB.RescheleitD.2001Comparison of different particles and methods for magnetic isolation of circulating tumor cells, Journal of Magnetism and Magnetic Materials, 225175179
115. SimioniA. R.PrimoF. L.RodriguesM. M. A.LacavaZ. G. M.MoraisP. C.TedescoA. C.2007Preparation, Characterization and in vitro Toxicity Test of Magnetic Nanoparticle-Based Drug Delivery System to Hyperthermia of Biological Tissues, IEEE Transactions on Magnetics, 4324592461
116. SinghN.JenkinsG. J. S.NelsonB. C.MarquisB. J.MaffeisT. G. G.BrownA. P.WilliamsP. M.WrightC. J.DoakS. H.2012The role of iron redox state in the genotoxicity of ultrafine superparamagnetic iron oxide nanoparticles, Biomaterials, 33163170
117. SkumielA.2006Suitability of water based magnetic fluid with CoFe2O4 particles in hyperthermia, Journal of Magnetism and Magnetic Materials, 3078590
118. SuW.WangH.WangS.LiaoZ.KangS.PengY.HanL.ChangJ.2012PEG/RGD-modified magnetic polymeric liposomes for controlled drug release and tumor cell targeting, International Journal of Pharmaceutics, 426170181
119. SunY.ChenZ.YangX.HuangP.ZhouX.DuX.2009Magnetic chitosan nanoparticles as a drug delivery system for targeting photodynamic therapy, Nanotechnology, 20135102135110
120. ThunemannA. F.SchuttD.KaufnerL.PisonU.MohwaldH.2006Maghemite Nanoparticles Protectively Coated with Poly(ethylene imine) and Poly(ethylene oxide)-block-poly(glutamic acid), Langmuir, 2223512357
121. TungW. L.HuS. H.LiuD. M.2011Synthesis of nanocarriers with remote magnetic drug release control and enhanced drug delivery for intracellular targeting of cancer cells, Acta Biomaterialia, 728732882
122. UrbinaM. C.ZinovevaS.MillerT.SabliovC. M.MonroeW. T.KumarC. S. S. R.2008Investigation of Magnetic Nanoparticle-Polymer Composites for Multiple-controlled Drug Delivery, The Journal of Physical Chemistry C, 1121110211108
123. Van der ZeeJ.2002Heating the patient: a promising approach?, Annals of Oncology, 1311731184
124. VeisehO.GunnJ. W.ZhangM.2010Design and fabrication of magnetic nanoparticles for targeted drug delivery and imaging, Advanced Drug Delivery Reviews, 62284304
125. VermisoglouE. C.PilatosG.RomanosG. E.DevlinE.KanellopoulosN. K.KaranikolosG. N.2011Magnetic carbon nanotubes with particle-free surfaces and high drug loading capacity, Nanotechnology, 22355602355612
126. VoitW.KimD. K.ZapkaW.MuhammedM.RaoK. V.2001Magnetic behavior of coated superparamagnetic iron oxide nanoparticles in ferrofluids, Materials Research Society Symposium Proceedings, 676Y7Y7.8.6.
127. WangH.WangS.LiaoZ.ZhaoP.SuW.NiuR.ChangJ.2012Folate-targeting magnetic core-shell nanocarriers for selective drug release and imaging, International Journal of Pharmaceutics, 430342349
128. WangL.NeohK. G.KangE.T.ShuterB.2011Multifunctional polyglycerol-grafted Fe3O4@SiO2 nanoparticles for targeting ovarian cancer cells, Biomaterials, 3221662173
129. WinerJ. L.LiuC. Y.ApuzzoM. L. J.2011The Use of Nanoparticles as Contrast Media in Neuroimaging: A Statement on Toxicity, World Neurosurgery, DOI:10.1016/j.wneu.2011.08.013,impress.
130. WuH.LiuG.WangX.ZhangJ.ChenY.ShiJ.YangH.HuaH.YangS.2011Solvothermal synthesis of cobalt ferrite nanoparticles loaded on multiwalled carbon nanotubes for magnetic resonance imaging and drug delivery, Acta Biomaterialia, 734963504
131. WustP.HildebrandtB.SreenivasaG.RauB.GellermannJ.RiessH.FelixR.SchlagP. M.2002Hyperthermia in combined treatment of cancer, The Lancet Oncology, 3487497
132. WustP.HildebrandtB.SreenivasaG.RauB.GellermannJ.RiessH.FelixR.SchlagP. M.2002Hyperthermia in combined treatment of cancer. The Lancet Oncology 3487497
133. YallapuM. M.OthmanS. F.CurtisE. T.GuptaB. K.JaggiM.ChauhanS. C.2011Multi-functional magnetic nanoparticles for magnetic resonance imaging and cancer therapy, Biomaterials, 3218901905
134. YangF.FuD. L.LongJ.NiQ. X.2008Magnetic lymphatic targeting drug delivery system using carbon nanotubes, Medical Hypotheses, 70765767
135. YangF.JinC.YangD.JiangY.LiJ.Di Y.HuJ.WangC.NiQ.FuD.2011Magnetic functionalised carbon nanotubes as drug vehicles for cancer lymph node metastasis treatment, European Journal of Cancer, 4718731882
136. YangJ.LeeH.HyungW.ParkS.B.HaamS.2006Magnetic PECA nanoparticles as drug carriers for targeted delivery: Synthesis and release characteristics, Journal of Microencapsulation, 23203212
137. YangQ.LiangJ.HanH.2009Probing the Interaction of Magnetic Iron Oxide Nanoparticles with Bovine Serum Albumin by Spectroscopic Techniques, The Journal of Physical Chemistry B, 1131045410458
138. YangX.HongH.GrailerJ. J.RowlandI. J.JavadiA.HurleyS. A.XiaoY.YangY.ZhangY.NicklesR. J.CaiW.SteeberD. A.GongS.2011cRGD-functionalized, DOX-conjugated, and ??Cu-labeled superparamagnetic iron oxide nanoparticles for targeted anticancer drug delivery and PET/MR imaging, Biomaterials, 3241514160
139. YangY.JiangJ. S.DuB.GanZ. F.QianM.ZhangP.2009Preparation and properties of a novel drug delivery system with both magnetic and biomolecular targeting, Journal of Materials Science: Materials in Medicine, 20301307
140. YaoA.AiF.WangD.HuangW.ZhangX.2009Synthesis, characterization and in vitro cytotoxicity of self-regulating magnetic implant material for hyperthermia application, Materials Science and Engineering C, 2925252529
141. YellenB. B.ForbesZ. G.HalversonD. S.FridmanG.BarbeeK. A.ChornyM.2005 Targeted drug delivery to magnetic implants for therapeutic applications, Journal of Magnetism and Magnetic Materials, Vol.293, pp.647-654.
142. YildirimerL.ThanhN. T. K.LoizidouM.SeifalianA. M.2011Toxicological considerations of clinically applicable Nanoparticles, Nano Today, 6585607
143. ZefengX.GuobinW.KaixiongT.JianxingL.YuanT.2005Preparation and acute toxicology of nano-magnetic ferrofluid, Journal of Huazhong University of Science and Technology-- Medical Sciences, 255961
144. ZhangL.ZhangF.WangY.S.SunY.L.DongW.F.SongJ.F.HuoQ.S.SunH.B.2011Magnetic colloidosomes fabricated by Fe3O4-SiO2 hetero-nanorods, Soft Matter, 773757381
145. ZhangY. Q.LiL. L.TangF.RenJ.2006Controlled Drug Delivery System Based on Magnetic Hollow Spheres/Polyelectrolyte Multilayer Core-Shell Structure, Journal of Nanoscience and Nanotechnology, 632103214
146. ZhouH.TaoK.DingJ.ZhangZ.SunK.ShiW.2011A general approach for providing nanoparticles water-dispersibility by grinding with poly (ethylene glycol). Colloids and Surfaces A: Physicochemical and Engineering Aspects 2011; 3891826
- 上一篇:鐵磁流體的用途 2023/11/4
- 下一篇:兒茶酚胺及其代謝物檢測:部分腫瘤的檢測標(biāo)記物 2023/11/3